DIS-16-04, Small Modular Reactors: Regulatory Strategy, Approaches and Challenges
Preface
Discussion papers play an important role in the selection and development of the regulatory framework and regulatory program of the Canadian Nuclear Safety Commission (CNSC). They are used to solicit early public feedback on CNSC policies or approaches.
The use of discussion papers early in the regulatory process underlines the CNSC's commitment to a transparent consultation process. The CNSC analyzes and considers preliminary feedback when determining the type and nature of requirements and guidance to issue.
Discussion papers are made available for public comment for a specified period of time. At the end of the first comment period, CNSC reviews all public input, which is then posted for feedback on the CNSC website for a second round of consultation.
The CNSC considers all feedback received from this consultation process in determining its regulatory approach.
Due to the broad scope of the subject matter, the CNSC recognizes that the readers of this discussion paper will be quite diverse, ranging from technology developers with a technical interest through decision and policy makers and to interested members of the public. Each group's level of familiarity with the Canadian regulatory approach may vary. To address this, this paper is structured with supplementary information included as appendices.
Table of Contents
- Executive Summary
- 1. Introduction
-
2. Key Regulatory Challenges
- 2.1 Introduction
- 2.2 Technical information, including research and development activities used to support a safety case
- 2.3 Licensing process for multiple module facilities on a single site
- 2.4 Licensing approach for a new demonstration reactor
- 2.5 Licensing process and environmental assessments for fleets of small modular reactors
- 2.6 Management system considerations: Licensees of activities involving small modular reactors
- 2.7 Safeguards implementation and verification
- 2.8 Deterministic/probabilistic safety analyses
- 2.9 Defence in depth and mitigation of accidents
- 2.10 Emergency planning zones
- 2.11 Transportable reactor concepts
- 2.12 Increased use of automation for plant operation and maintenance
- 2.13 Human/machine interfaces in facility operation
- 2.14 The impact of new technologies on human performance
- 2.15 Financial guarantees for operational continuity
- 2.16 Site security provisions
- 2.17 Waste management and decommissioning
- 2.18 Subsurface civil structures important to safety
- 3. Fusion technologies
- 4. Conclusion
- 5. How to participate
- Appendix A : Overview of Design Concepts for Small Modular Reactors
-
Appendix B : Additional Background Information
- B.1 Background
- B.2 Additional information about information to support a safety case
- B.3 Current licensing practice for multiple reactor facilities on a single site
- B.4 Additional information on licensing considerations for new demonstration reactors
- B.5 Background on licensing process and environmental assessments for fleets of SMRs
- B.6 Additional information on licensee management system considerations for projects involving small modular reactors
- B.7 Background information on safeguards verification
- B.8 Additional information about deterministic and probabilistic safety analyses
- B.9 Additional information on defence in depth and mitigation of accidents
- B.10 Background on emergency planning zones
- B.11 Additional information on increased use of automation for plant operation and maintenance
- B.12 Background on waste management and decommissioning
- B.13 Background on sub-surface civil structures important to safety
- Appendix C : Consolidation of questions
- Appendix D : Specific Drivers for Regulatory Readiness
Executive Summary
Nuclear reactors of varying sizes and power outputs have been used for a variety of applications, such as research, materials testing, medical uses, and electrical power generation. The CNSC regulates activities associated with all of these applications.
In recent years, novel reactor technologies have emerged to supply power to smaller electrical grids or remote, off-grid areas. These technologies are commonly referred to as small modular reactors (SMRs). Designers claim that SMRs will be able to service regions where larger nuclear power plants (NPP) are not practical or possible.
Electrical utilities, industry groups and government agencies throughout the world are investigating alternative uses for SMRs beyond electricity generation. These include producing steam supply for industrial applications and district heating systems, and producing value-added products such as hydrogen fuel and desalinated drinking water.
To keep current on technology trends and regulatory implications, many nuclear regulators, including the CNSC, have been reviewing the application of novel features in more traditional, and larger, NPP designs. SMRs, however, represent novel technologies that aim to achieve greater efficiencies and reduced operational costs. One of the key questions that nuclear regulators – including the CNSC – must address with vendors and other stakeholders is what are the regulatory and licensing implications presented by SMRs? If a proponent decides to deploy such technologies in Canada, what are some of the key regulatory issues that need to be resolved in advance to meet Canadian licensing requirements?
The Canadian regulatory approach is built on a long-established foundation of risk-informed regulationFootnote [1]. Regulatory tools and decision-making processes are structured to enable a licence applicant for a reactor facility to propose alternative ways to meet safety objectives. CNSC requirements set safety performance objectives that applicants must meet and these objectives can be achieved in various ways. However, proposals must demonstrate, with suitable information, that they are equivalent to or exceed regulatory requirements. CNSC requirements and guidance for reactor facilities are generally articulated to be technology neutral and where possible permit the use of the graded approach. The graded approach enables applicants to establish the stringency of design measures, safety analyses and provisions for conduct of their activities commensurate with the level of risk posed by the reactor facility.
CNSC tools for assessment, decision making, compliance verification and enforcement ensure that approaches proposed in a licence application will prevent unreasonable risk – to the environment, to the health and safety of persons and to national security – associated with the development, production, possession or use of those technologies.
Regarding the regulations and licensing process in Canada, it is the CNSC's view that:
- Existing regulations under the Nuclear Safety and Control Act (NSCA) are already suitable for regulating activities involving the use of SMR technologies.
- The licensing process is risk-informed and independent of reactor technology or size, but CNSC is interested in understanding where enhancements can be made.
- Licence applications for specific activities and project phases can be reviewed in series or in parallel depending on the needs and the readiness of the applicant.
Even with a flexible regulatory approach, some innovative features may present challenges in both interpretation and application of requirements. The CNSC has examined a number of key areas where potential challenges could exist around emerging technologies, such as SMRs. In some cases, the CNSC is able to confirm that the existing requirements remain valid and useful. In a number of other areas, the implications of innovative approaches need to be examined to a greater degree to confirm whether additional requirements or guidance are needed to further support those already existing.
This discussion paper provides an overview of a number of the potential issues associated with SMRs that the CNSC has examined. These issues are described at a high level along with a short description of specific items to be addressed in future work. In other cases, the paper presents how the CNSC plans to address the issues using existing regulatory tools and processes.
The CNSC would like to hear from all interested stakeholders on the topics addressed in this paper as well as any others relevant to the discussion on SMRs.
1. Introduction
Over the past several years, a number of technology developers have expressed interest in the possible construction and deployment of small modular reactors (SMRs) in Canada. They have sought to understand how the Canadian Nuclear Safety Commission (CNSC) is establishing a state of readiness to regulate activities that would utilize SMRs. Canadian government agencies, science and technology institutions, utilities, industry associations, other nuclear regulators and interested members of the public have also expressed interest in the CNSC's readiness preparations.
New technologies being developed (which include SMRs and advanced reactors) vary significantly in size, design features and cooling types. In addition, the locations at which they might be sited could potentially be different from past nuclear power plant (NPP) projects. For example, they could be located:
- on small grids where power generators need to remain below, for example, 300 megawatt electric (MWe) per facility to maintain grid stability
- at edge-of-grid or off-grid locations where power needs are small – in the range of 2 to 30 MWe – but where energy production is currently very expensive and dependent on fossil fuel
Alternative uses, beyond electricity generation, are also being considered. These include steam supplies for industrial applications and district heating systems, and the production of value-added products such as hydrogen fuel or desalinated drinking water.
Appendix A provides an overview of SMR design concepts and a list of SMR vendors that have requested regulatory information from the CNSC.
Most SMR concepts, although based on technological work and operating experience from past and existing plants, propose to employ a number of novel approaches. Novel approaches can affect the certainty of how the plant will perform under not only normal operation, but also in accident conditions, in which predictability is paramount to safety. These novel approaches and their corresponding uncertainties raise regulatory questions during the licensing process.
The Canadian regulatory approach is built on a long-established foundation of risk-informed regulation. Regulatory tools and decision-making processes are structured to enable a licence applicant for a reactor facility to propose alternative ways to meet regulatory expectations. Proposals must demonstrate, with suitable information, that they are equivalent to or exceed regulatory requirements. Current requirements and guidance for reactor facilities are generally articulated to be technology neutral. Where possible, they permit the use of a graded approach that enables applicants to establish the stringency of design measures, safety analyses and provisions for conduct of their activities commensurate with the level of risk posed by the reactor facility. Factors to be considered in the graded approach include:
- reactor power
- source term
- amount and enrichment of fissile and fissionable material
- spent fuel elements, high pressure systems, heating systems and the storage of flammables, which may affect the safety of the reactor
- type of fuel elements
- type and the mass of moderator, reflector and coolant
- amount of reactivity that can be introduced and its rate of introduction, reactivity control and inherent and additional features
- quality of the confinement structure or other means of confinement
- utilization of the reactor
- siting, which includes proximity to population groups or extent of isolation from emergency responders
All of these factors have been considered in past licensing and compliance activities for Class I facilities such as prototype reactors (e.g., Whiteshell WR-1), demonstration reactors (e.g., Rolphton Nuclear Power Demonstration and Douglas Point Nuclear Generating Station), research reactors and Canadian fuel cycle facilities.
However, in view of novel approaches, CNSC staff are reviewing:
- some of the key regulatory issues that may need to be resolved to meet Canadian licensing requirements
- the adequacy of existing regulatory framework tools to address potential near- and long-term projects
- potential changes to regulatory framework tools to ensure novel approaches appropriately consider safety
The CNSC has examined a number of key areas where potential challenges could exist. In some cases, the CNSC is able to confirm that the existing requirements remain valid and useful. In a number of other areas, implications of the innovative approaches need to be examined to a greater degree to confirm the level of applicability of existing requirements and guidance – and whether different requirements or guidance are needed to further support those already existing.
The CNSC will consider feedback from stakeholders on the discussion paper in ongoing updates to its regulatory framework.
2. Key Regulatory Challenges
2.1 Introduction
Most small modular reactor (SMR) concepts, although based on technological work and operating experience from past and existing plants, employ a number of novel approaches simultaneously. Novel approaches can affect the certainty of how the plant will perform under not only normal operation but also under accident conditions, in which predictability is paramount to safety.
In addition to addressing the technical challenges of designing an SMR, an SMR proponent also needs to ensure that the design meets the CNSC's regulatory requirements.
This section examines some key areas where novel approaches may present uncertainties, and where information from the public and interested stakeholders would help inform regulatory policy. The following list of topics was developed based on approximately five years of interaction with SMR vendors, utilities, government agencies and other interested stakeholders who have stated that these are important to the discussion. The topics are:
- technical information, including research and development activities used to support a safety case
- licensing process for multiple module facilities on a single site
- licensing approach for a new demonstration reactor
- licensing process and environmental assessments for fleets of SMRs
-
management system considerations
- licensees of activities involving SMRs
- safeguards verification
- deterministic and probabilistic safety analyses
- defence-in-depth and mitigation of accidents
- emergency planning zones
- transportable reactor concepts
- increased use of automation for plant operation and maintenance
- human/machine interfaces in facility operation
- impact of new technologies on human performance
- financial guarantees for operational continuity
- site security provisions
- waste management and decommissioning
- subsurface civil structures important to safety
Appendix B provides additional information to supplement the reader's understanding of the fundamentals and rationale for regulation of the topics listed above. The main audience for this information includes people who are less familiar with the Canadian approach to regulation and who want to understand why these areas are important for safety. This information does not establish any new regulatory policy and has been compiled from diverse information located throughout the CNSC's existing regulatory framework.
2.2 Technical information, including research and development activities used to support a safety case
Technical information such as systematic industry research and development activities plays a crucial role in supporting the credibility of safety claims made by an applicant both in the licensing process and during conduct of licensed activities. This information adds to the understanding of risks associated with proposed activities (e.g., operation of a reactor facility) and assists in demonstrating, with high confidence, that appropriate control measures are in place.
SMR vendors claim that their proposed designs are simpler, and that they incorporate economy of mass production, enhanced engineered security features and a high level of passive or inherent safety in the event of malfunctions and accidents. The proposed designs combine safety approaches in multiple interfacing plant systems that involve:
- new materials, fuels and fabrication techniques
- alternative construction approaches
- passive features with either minimal or limited operating experience (in some cases, experience may come from experiments conducted decades ago)
- new human/machine interface concepts and levels of automation (including methods to address potential for human error)
- operating approaches such as potential deep load-followingFootnote [2] (NPPs were traditionally base-load facilities) that may affect aging mechanisms, as well as operating and safety margins
- new or enhanced computer codes used to simulate and predict the behaviours of the engineered systems under operational and accident conditions
- resolution of applicable safety issues from existing reactor designs
- alternative methods of design and safety assessment
- alternative approaches for maintenance and reliability
All of these approaches require quality assured and credible information that is supported by research and development in order to demonstrate claims of safety. In many cases, regulatory authorities have not yet reviewed these claims. In others, there may be significant gaps in information between past experiments (in prototypes and demonstration facilities) and information needed to support long-term operation of a modern power plant.
CNSC regulatory document RD/GD-369, Licence Application Guide: Licence to Construct a Nuclear Power Plant, outlines the level of information necessary to support safety claims in an application for a construction licence. Applicable regulatory documents for the safety and control area further expand on the scope and depth of supporting information.
The CNSC pre-licensing vendor design review process, as described in GD-385, Pre-licensing Review of a Vendor's Reactor Design, provides an early opportunity for a reactor vendor to demonstrate that it has a research and development program in place that is adequate to support a future safety case, and in particular, to demonstrate the safety adequacy of any novel aspects of facility design or operation.
The CNSC evaluates how well licensees meet regulatory requirements and expectations for the performance of programs in 14 safety and control areas (SCAs). SCAs are the technical topics used by the CNSC to access, review, verify and report on regulatory requirements and performance across all regulated facilities activities. They are grouped according to their functional areas (management, facility and equipment, and core control process) as follows:
-
management
- management systems
- human performance management
- operating performance
-
facility and equipment
- safety analysis
- physical design
- fitness for service
-
core control processes
- radiation protection
- conventional health and safety
- environmental protection
- emergency management and fire protection
- waste management
- security
- safeguards
- packaging and transport
For the topic of "technical information, including research and development activities used to support a safety case", are requirements regarding the scope and adequacy of supporting information sufficiently clear?
Of particular interest are whether existing R&D requirements are clear in key regulatory documents such as REGDOC-2.5.2, Design of Reactor Facilities: Nuclear Power Plants, RD-367, Design of Small Reactor Facilities, REGDOC-2.4.1, Deterministic Safety Analysis, and other documents related to facility lifecycle (e.g., REGDOC-2.6.3, Fitness for Service: Aging Management).
2.3 Licensing process for multiple module facilities on a single site
The Canadian licensing process (see REGDOC-3.5.1, Licensing Process for Class I Nuclear Facilities and Uranium Mines and Mills) under the Nuclear Safety and Control Act (NSCA) addresses the applicant's proposed activities.
If an applicant proposes to construct and operate a facility, all of the activities associated with the proposal will be considered in the licence application, including construction and operation of multiple modules (or units) on a single site. The NSCA gives the Commission the flexibility to encompass all activities either under one licence or multiple licences depending on the nature and timelines of the proposed activities. The applicant must demonstrate that its proposal meets the requirements applicable to the proposed activities. The CNSC already has a number of licensees with multiple reactors operating under a single licence.
Through licence application guides (LAGs) such as RD/GD-369, Licence Application Guide: Licence to Construct a Nuclear Power Plant, and regulatory requirements and guidance articulated in regulatory documents such as REGDOC-2.5.2, Design of Reactor Facilities: Nuclear Power Plants, and RD-367, Design of Small Reactor Facilities, the CNSC expects the safety case to address multiple unit accidents, and requirements at the facility level.
The CNSC is aware that a small number of reactor developers are developing reactors with replaceable reactor core modules. Beyond CANDU refurbishment activities (which replaces a limited number of reactor components), there is no regulatory precedent in Canada for the complete replacement of reactor vessels in a nuclear power plant (NPP)Footnote [3].
Also being developed in other parts of the world are marine-based floating and submersible power plants, with multiple reactors that could be relocated from one potential site to another. Currently the environmental assessment and licensing processes consider only a single deployment site. No Canadian precedent exists for licensing of activities related to this type of facility.
For the topic of "Licensing process for multiple module facilities on a single site", are clarifications needed to REGDOC-3.5.1, Licensing Process for Class I Nuclear Facilities and Uranium Mines and Mills?
In order to be better prepared for the use of replaceable reactor core modules or relocatable facilities, the CNSC is seeking information on facility deployment strategies being considered by developers, including impacts of such an approach on areas such as worker and public safety, environmental assessment and decommissioning.
The CNSC will use this information for future more-detailed workshops to discuss regulatory implication of different deployment approaches.
2.4 Licensing approach for a new demonstration reactor
Nuclear demonstration facilities are not new to Canada and have included the Rolphton Nuclear Power Demonstration project, Douglas Point Nuclear Generating Station and the Gentilly-1 Nuclear Generating Station. However, initial licensing of this kind of facility has not been performed in Canada since the late 1960s.
A demonstration reactor facility can be thought of as a full-scale and, for the most part, a fully functional integrated facilityFootnote [4] with features that enable the collection of operating experience to further address uncertainties associated with novel approaches.
Many companies have asked if the CNSC has a simplified licensing approach for demonstration facilities. A demonstration reactor facility is a Class 1A nuclear facility and therefore subject to the same regulations as a full-scale nuclear power plant.
The licensing process for a demonstration facility is the same as that of any other reactor facility. It is described in REGDOC-3.5.1, Licensing Process for Class I Nuclear Facilities and Uranium Mines and Mills. Licensing is focused on the proposed activities irrespective of the technology used.
The starting point for an application for a demonstration facility licence will be the existing CNSC licence application guides such as RD/GD-369, Licence Application Guide Licence to Construct a Nuclear Power Plant. As mentioned earlier, the fundamental information needed for a successful demonstration facility licence application remains the same as that of any other reactor facility. The licence application guides speak clearly to the need for high quality information to support submissions for each safety and control area in the application. The existing requirements in regulatory documents for each safety and control area take the graded approach into account. Any uncertainties in the safety and control measures might influence the application of the graded approach. In some cases, a demonstration facility would be required to have additional safety and control measures to mitigate risk.
For the topic of "Licensing approach for a new demonstration reactor", is there a need for additional clarification or information beyond that found in RD/GD-369, Licence Application Guide: Licence to Construct a Nuclear Power Plant? If yes, what needs to be clarified or added?
With respect to addressing uncertainties introduced by the application of integrated multiple novel features in a demonstration facility, are requirements regarding the scope and adequacy of supporting information sufficiently clear?
What, if any, requirements need to be revisited to address activities involving demonstration reactors? For example, are additional requirements or guidance needed to address operational restrictions if the facility is being used to gather operating experience that would be normally be needed for commercial facility licences?
2.5 Licensing process and environmental assessments for fleets of small modular reactors
As discussed in section 2.3, for edge-of-grid or even off-grid applications in remote parts of Canada, the CNSC is aware that a number of vendors are considering SMR concepts in the per-unit range of 3 to 25 MWe, depending on customers' power needs. Vendors are considering these projects as either supplementary to an existing northern grid-system or as an off-grid source. Some of these vendors are considering a business model in which the vendor would also own and operate such facilities. This proposed plan sets the stage for a fleet of small similar – if not identical – facilities across a wide geographical area.
REGDOC-3.5.1, Licensing Process for Class I Nuclear Facilities and Uranium Mines and Mills, provides an overview of the licensing process for Class I nuclear facilities and uranium mines and mills in Canada, taking into consideration the requirements of the NSCA and associated regulations.
In accordance with paragraph 13 of the Canadian Environmental Assessment Act, 2012 (CEAA), an SMR project, fleet or otherwise, would be subject to an environmental assessment (EA) that considers and evaluates potential adverse environmental effects. On lands where the CEAA applies, the CNSC is the sole federal responsible authority for conducting the EA. As per the CEAA, an EA includes information prepared by the CNSC and comments received from Aboriginal groups and the public. The results from this EA form a benchmark against which any future work will be compared.
For applicants proposing an SMR project in areas of Canada with a land claim agreement where the CEAA does not apply, CNSC staff will support the EA process of that land claim regime. Further, the CNSC will use the information gathered in the EA process to inform its licensing decision under the NSCA. Questions have arisen about how licensing and environmental assessments might be conducted for fleet deployments over a large geographical area where the owner-operator is the same entity, the technology remains the same across the fleet, and the physical environment of all sites fits within a predefined set of bounding environmental effects parameters.
For the topic of "licensing process and environmental assessments for fleets of SMRs", how do you envision proposals for such fleets across large geographical territories proceeding through licensing and environmental assessments?
How would the principles discussed in REGDOC-3.5.1, Licensing Process for Class I Nuclear Facilities and Uranium Mines and Mills be applied and where might challenges exist?
2.6 Management system considerations: Licensees of activities involving small modular reactors
Management systems are used to bring together – in a planned and integrated manner – the processes necessary to ensure safe conduct of licensed activities, as well as to achieve other goals such as sustainability and business success.
The General Nuclear Safety and Control Regulations and the Class I Nuclear Facilities Regulations contain requirements for information to be submitted in licence applications for reactor facilities. The information must demonstrate that the licensee will have adequate programs and controls in place to safely conduct the nuclear activities for which the licence is sought. Requirements and guidance for most SCAs outline specific requirements applicable to the applicant's management system. The practice in Canada is that the entity overseeing the licensed activities must be the licensee. For an operating facility, this would be the operator.
The CNSC licence application guide for construction, RD/GD-369, Licence Application Guide: Licence to Construct a Nuclear Power Plant, as well as specific regulatory documents, set requirements and guidance for each SCA and for management system processes and procedures necessary to support the safety case in a licence application. In particular, licensees are expected to demonstrate that they comply with CSA Group's (formerly called Canadian Standards Association Group) standard N286-12, Management system requirements for nuclear facilities. This includes, for example, management system processes for oversight of contractors and the ongoing adequacy of supporting research and development information.
SMR developers are implementing approaches that seek to standardize design, manufacturing and onsite installation of plant modules. In the future, operators will be expected to consider the implications of this approach in their management systems, including provisions such as ongoing access to the technical information to support the safety case. For example, the applicant will have to disposition any codes and standards used by the manufacturer. In some cases, such as the use of sealed reactor modules, it may be difficult to conduct receipt inspections in order to permit the module to be installed and placed in service. The CNSC has articulated requirements for construction and commissioning programs in REGDOC-2.3.1, Conduct of Licensed Activities: Construction and Commissioning Programs.
In the Canadian nuclear industry, all current power plants are owned by provincial governments and operated by utilities, which in turn may be Crown corporations or private companies. The CNSC is aware that applicants for SMR projects may seek to employ alternative models such as involving substantial investment from offshore sources for build-own-operate approaches. For example, a number of vendors of very small power plants for off-grid use have indicated an interest in pursuing build-own-operate models for a number of practical reasons. It is important to understand that this is not an SMR-specific issue but rather a trend that may emerge first in Canada through SMR deployment.
For the topic of "Management system considerations: Licensees of activities involving SMRs", to help the CNSC prepare for alternative ownership and operating models that would be used in SMR deployment, more details (such as case studies) are sought regarding areas including:
- how deployment of different SMR concepts (e.g., factory fueled transportable concepts) would proceed
- how oversight for such deployments would be conducted
- how issues such as licensee performs inspections of key components (e.g., a reactor module) when received from a vendor
- how alternative ownership models will address requirements in CSA Group's standard N286‑12, Management system requirements for nuclear facilities and in CNSC regulatory requirements
The CNSC will use this information for future, more detailed workshops to discuss regulatory implication of
different deployment approaches.
See section B.6 of appendix B for additional background information.
2.6.1 Management system: Minimum complement in small modular reactor facilities
The primary driver behind minimum complement in the nuclear facility is the safety case, taking into account anticipated plant states.
The minimum complement is the minimum number of qualified workers who must be present at all times to ensure the safe operation of the nuclear facility and to ensure adequate emergency response capability.
Having a minimum complement of qualified personnel is an important part of ensuring safe conduct of activities during normal and abnormal conditions. A minimum complement is not only determined by design characteristics, but also by the characteristics of each site and the surrounding region.
All Class I nuclear facility licensees are required to ensure the presence of a sufficient number of qualified workers to carry on the licensed activity safely and in accordance with the NSCA, the regulations made under the NSCA, and the facility licence. The CNSC licence application guide for construction (RD/GD-369, Licence Application Guide: Licence to Construct a Nuclear Power Plant) presents the information required of an applicant regarding minimum complement.
CNSC regulatory document G-323, Ensuring the Presence of Sufficient Qualified Staff at Class I Nuclear Facilities – Minimum Staff Complement articulates the risk-informed approach that a licence-applicant can use. The document also speaks to both single unit and multiple unit facilities. The guidance is flexible, so applicants can apply it to different sizes and types of SMRs.
A number of those workers specified in the minimum shift complement must be certified as per regulatory document RD-204, Certification of Persons Working at Nuclear Power Plants, which articulates requirements that must be met. Those requirements would apply to activities that involve the use of SMRs.
A number of SMR developers are seeking to develop technologies that reduce the need for onsite human support in a facility, such as:
- instrumentation and control architectures to replace the need for field surveillance by onsite personnel
- reactor safety characteristics that reduce the need for human intervention or provide for long grace timesFootnote [5] under anticipated plant operating conditions
For the topic of "Management system: minimum complement in SMR facilities", are the regulatory requirements and guidance related to minimum complement sufficient and clear as applied to activities involving SMRs? What, if any, proposed changes should be considered for the existing regulatory requirements? For example, in conjunction with the question in section 2.12, is additional guidance needed to address human coverage for failure of automated systems?
2.7 Safeguards implementation and verification
Safeguards are measures of international control that are applied by the CNSC and the International Atomic Energy Agency (IAEA) on nuclear material and activities, and through which the IAEA seeks to independently verify that nuclear facilities are not misused and nuclear material is not diverted from peaceful uses. States accept these measures through safeguards agreements.
In the CNSC regulatory framework, the safeguards and non-proliferation SCA covers the programs and activities required for the successful implementation of the obligations arising from the Canada/IAEA safeguards agreements as well as all other measures arising from the Treaty on the Non-Proliferation of Nuclear Weapons. Sections 12(1)(i) and 30(1) of the General Nuclear Safety and Control Regulations require the licensee to take all necessary measures to facilitate Canada's compliance with any applicable safeguards agreement, and defines reporting requirements for safeguards events, respectively.
Additional requirements for safeguards and non-proliferation include nuclear material accountancy and control as stipulated in RD‑336, Accounting and Reporting of Nuclear Material. The other three specific areas of requirements covered by this document include:
- access and assistance to the IAEA – licensees must provide access and assistance to the IAEA or the CNSC for inspection, verification, evaluation or other safeguards activities as needed
- safeguards equipment, containment and surveillance – licensees must facilitate the installation of IAEA equipment and support IAEA equipment operation, maintenance and upgrade, as necessary
- operational and design information – licensees must provide design information, operational information or records, as well as the annual update to the additional protocol declaration, to the CNSC
The types and characteristics of fissile materials in fuels and the fuel designs themselves proposed for use in SMR technologies are expected to vary widely from design to design. Materials and fuels could include:
- fuels and compositions already used in current NPP fleets
- liquid fuels (for molten salt reactors)
- metallic and graphite-based fuel concepts (for high-temperature gas and liquid metal reactors)
In some cases, the designs for safeguards may not be fully proven and require further development (e.g., liquid fuels such as those proposed for molten salt reactors).
Some SMR deployment scenarios will present specific technical and logistic challenges to safeguards inspections. For example, fleets of smaller SMR facilities distributed across a large and possibly remote geographical region make physical inspections complex from a travel perspective. As a result, alternative but equally rigorous approaches for such deployment scenarios may need to be developed to facilitate efficient and effective inspections. One example proposed by vendors involves using specially designed but tamper-proof instruments that could communicate information remotely to safeguards. This is called safeguards by design, which is supported in RD‑336, Accounting and Reporting of Nuclear Material. In the regime of safeguards by design, vendors are encouraged to communicate their designs to the CNSC and the IAEA at an early stage so that safeguards measures, requirements and equipment installations can be integrated into the facility designs and construction.
GD-385, Pre-licensing Review of a Vendor's Reactor Design, explains how the CNSC provides an opportunity for a reactor vendor to obtain early feedback to verify that the proposal meets Canadian requirements for the implementation of safeguards in the design. Focus area 15 of the vendor design review – robustness security and safeguards – confirms that the vendor's submitted documentation is consistent with Canada's overall safeguards approach and would facilitate Canada's obligations under its safeguards agreements with the IAEA. The vendor can use this information for discussion with potential customers who may apply for a CNSC licence to build and operate a reactor facility.
For the topic of "Safeguards implementation and verification", the CNSC would like to hear if its current framework provides enough clarity to effectively ensure safeguards verification of novel fuels and new designs.
2.8 Deterministic/probabilistic safety analyses
The purpose of safety analysis is to establish and confirm the design basis, derive operational limits and establish and validate accident and management procedures and guidelines. One of the objectives of the safety analysis is to demonstrate that the systems in an NPP can prevent unacceptable consequences of an event. Mitigating systems are usually identified with safety systems.
The requirements for safety analysis in support of an application for a licence are articulated in the Class I Nuclear Facilities Regulations.
Further requirements and guidance including considerations of the use of the graded approach are described in:
- REGDOC-2.4.1, Deterministic Safety Analysis
- REGDOC-2.4.2, Safety Analysis: Probabilistic Safety Assessment (PSA) for Nuclear Power Plants
Developers of new reactor technologies, such as SMRs, are proposing to introduce multiple levels of alternative and innovative features intended to simplify and improve accident prevention and mitigation. For example, proposed control strategies as well as the use of passive safety features are intended to reduce the need for operator intervention (time and scope of response) during events.
Also of importance to SMRs is that they may be sited as multiple unit facilities, and the safety analysis needs to take this into account.
Uncertainties presented by alternative and innovative features can and do affect the outcomes of safety analyses.
For the topic of "deterministic/probabilistic safety analyses", are the regulatory requirements and guidance clear for the kinds of alternatives that might be proposed for Deterministic/probabilistic safety analyses for SMR facilities? Do the existing requirements permit the establishment of a suitable level of probabilistic safety analysis for different novel designs?
Does enough information currently exist to apply probabilistic safety analysis to novel designs?
2.9 Defence in depth and mitigation of accidents
The CNSC is aware that designers of new reactor technologies, including SMRs, may propose alternative approaches to address the levels of defence in depth. For example, they may propose different physical barriers to be used. The CNSC requires all levels of defence in depth to be addressed in a safety case. These levels are described in general terms below.
Level 1
- Prevent deviations from normal operation, and prevent failures of structures, systems and components important to safety.
Level 2
- Detect and intercept deviations from normal operation, in order to prevent anticipated operational occurrences from escalating to accident conditions and to return the plant to a state of normal operation.
Level 3
- Minimize the consequences of accidents by providing inherent safety features, fail-safe design, additional equipment and mitigating procedures.
Level 4
- Ensure that radioactive releases caused by severe accidents are kept as low as practicable.
Level 5
- Mitigate the radiological consequences of potential releases of radioactive materials that may result from accident conditions.
Defence in depth is an integrated concept applied throughout the operation and design of a nuclear facility to provide levels of defence aimed at preventing accidents and ensuring appropriate protection in the event that prevention fails. This concept, as depicted in appendix B.9, Additional information on defence in depth and mitigation of accidents, enables the operator to detect, correct or compensate for a failure particularly to:
- prevent a failure from propagating to a higher level of defence
- mitigate consequences of a failure from a lower level of defence
- consider organizational and human performance
The principles of defence in depth, which play a large role in nuclear safety, are reflected in CNSC requirements and guidance and are expected to be addressed in all activities involving nuclear reactors, regardless of facility size and technology type.
The CNSC's regulatory framework details requirements and guidance with respect to implementing defence in depth approaches. The following chart lists key regulatory documents.
Area | CNSC specific requirements and guidance concerned with defence in depth |
---|---|
Addressing defence in depth in physical design |
Defence in depth framework and basis:
|
Assessing adequacy of defence-in-depth levels |
|
Management of accidents |
In addition, there are several CSA Group standards that support the requirements listed above in key program areas.
For the topic of "defence in depth and mitigation of accidents", given some of the novel safety approaches that vendors are proposing, are the existing requirements and guidance around defence in depth adequately clear for prevention and mitigation of accidents? Consider this question with particular attention to the following topics and combinations thereof:
- application of inherent and/or passive safety features
- application of alternative instrumentation and control strategies (e.g., remote monitoring and intervention of a fully-automated facility)
- non-water cooled technologies
- transportable sealed and factory fueled SMRs (see section 2.11)
- facilities proposed to be located in highly remote regions
2.10 Emergency planning zones
Technology developers are seeking ways to reduce emergency planning zones (EPZ) size, taking into account technology improvements.
Appendix B, section B.10 defines EPZs and discusses the factors to be considered in establishing them. Offsite emergency response measures, applied to each EPZ, are considered to be Level 5 of defence in depthFootnote [6]. More importantly, such measures are part of an integrated accident management approach that works in concert with defence in depth Levels 2 to 5. (See section 2.9 for further information.)
There are no legislative or regulatory requirements for EPZ sizing in Canada and therefore no restrictions currently in place on minimum EPZ size. EPZ and other planning actions should be undertaken in relation to the risks associated with the specific technology. As such, results from safety analyses (i.e., the probabilistic safety analysis) in combination with the protection strategy used by offsite planners will determine the EPZ size. This is consistent with the overall methodologies documented by the IAEA.
The CNSC provides requirements and guidance for applicants on key areas that factor into the methodology for determining the extent of EPZs – areas such as physical design of reactor facilities (REGDOC-2.5.2, Design of Reactor Facilities: Nuclear Power Plants, and RD-367, Design of Small Reactor Facilities) and safety analysis (REGDOC-2.4.1, Deterministic Safety Analysis, and REGDOC-2.4.2, Safety Analysis: Probabilistic Safety Assessment (PSA) for Nuclear Power Plants). This guidance also assists reactor vendors in the development of new reactor designs they are intending for Canadian applications. The Class I Nuclear Facilities Regulations require an application for a licence for a reactor facility to demonstrate that the selected design has accommodated specific site and regional characteristics. Composite bounding designs submitted as a bounding approach are possible; however, the applicant is limited to the projected releases as set in the environmental assessment and confirmed at the time of the construction licence review.
Site evaluation also plays a key role in the identification of postulated initiating events for the specific site. The CNSC provides requirements and guidance on site evaluation for new NPPs. In addition to the documents listed above, further information on site evaluation is contained in RD‑346, Site Evaluation for New Nuclear Power Plants. In the construction licence application, the estimates of releases and disturbances used in risk modelling are confirmed when the design and safety features of the NPP have been confirmed. The licensee is then expected to re-evaluate risk modelling as operating experience is gained over the facility lifetime. CNSC staff then review re-evaluated risk models as necessary.
As per REGDOC-2.10.1, Nuclear Emergency Preparedness and Response, applicants and licensees are required to provide offsite emergency planners with information such as results of safety analyses, which planners require to establish EPZs and plans. Applicants and licensees also must work with and support appropriate offsite organizations, such as provincial governments, to develop an effective onsite and offsite emergency response plan. This information influences decisions on the extent of an EPZ.
In addition to the above CNSC regulatory documents, the CSA Group also maintains standards that support and address areas relevant to information used to support cases for emergency planning.
For the topic of "emergency planning zones", are the requirements and guidance related to EPZs sufficiently clear to enable an organization to submit a licence application for a facility-specific EPZ while still meeting the CNSC's expectations regarding the environment and worker health and safety?
Are there specific considerations that need to be incorporated into requirements and guidance for specific siting cases like remote regions?
2.11 Transportable reactor concepts
The traditional model of reactor refuelling currently used at nuclear power plants around the world is to perform individual fuel element replacements at the facility site. Fresh fuel is delivered to the site in suitable packaging and spent fuel is kept on the site in safe storage following removal from the reactor. The fuel residence time in the reactor core is typically a couple of years (or in some cases less) before it is considered spent.
For edge-of-grid or even off-grid applications, a number of vendors are considering SMR concepts of approximately 3 to 35 MWe (per unit) using a compact nuclear core vessel that would either be entirely replaceable or would have its entire fuel inventory replaced in a manner similar to a fuel cartridge. Using this approach, operators intend to reduce or even eliminate lengthy refuelling operations at the deployment site and possibly facilitate quicker removal from the deployment site. The spent fuel inventory might then either be stored onsite or shipped to another location for refurbishment or disposal.
All shipments of nuclear substances in Canada are subject to the CNSC's Packaging and Transport of Nuclear Substances Regulations, 2015 (PTNSR 2015) as well as Transport Canada regulations. The CNSC issues certificates for certain types of packages and issues licences for certain types of shipments in accordance with the PTNSR 2015. These regulations are based on the IAEA's Regulations for the Safe Transport of Radioactive Material 2012 Edition. More information on regulation of the transport of nuclear substances, such as the potential need for a transport security plan and the potential certification of new or modified packages, can be found on the Packaging and transport of nuclear substances page of the CNSC website.
The approach for licensing all activities concerning the deployment of transportable reactor concepts and understanding how requirements would be met depends on the deployment scenario proposed and the nature of the activities in each phase of deployment. In particular:
- For the deployment site itself where the facility will be installed and operated (i.e., power plant location), the existing requirements and guidance for reactor facilities as well as licensing process would apply (e.g., REGDOC-3.5.1, Licensing Process for Class I Nuclear Facilities and Uranium Mines and Mills and RD-346, Site Evaluation for New Nuclear Power Plants).
- The licensing process for the facility handling new and spent fuel for a fleet of such transportable reactors would likely be very similar to that used for a spent fuel management facility which is a Class I facility. Requirements and guidance to be applied would be commensurate with the risks presented but might be very similar to those that would be applied to a small reactor project.
- Transport of new and spent fuel would be regulated using the PTNSR 2015.
For the topic of "Transportable reactor concepts", the CNSC is seeking information about deployment scenarios for further discussion. Examples of questions to inform future discussions include:
- How might deployment of such concepts proceed? (The CNSC seeks examples such as case studies.)
- What nature of activities will occur at the factory or service facility versus the site and how will those activities interface with one another from a management-system perspective?
- What would environmental impact statements look like?
- What would the relationship between the manufacturing facility, the facility fuelling the reactor modules, the carrier transporting the modules and the site operator entail?
- How would post-shipment inspections be conducted and addressed by the licensee of the deployment site facility?
- How would these scenarios be impacted if major components or modules were imported or exported?
- How would transport be conducted such that transport requirements would be met throughout the deployment journey?
- What is the strategy for performing safety analysis for all deployment activities?
2.12 Increased use of automation for plant operation and maintenance
The developers of SMR technology are seeking to increasingly automate as many operational and maintenance functions as possible to:
- improve precision and efficiencies of processes
- improve processes such as monitoring equipment reliability continuously rather than through periodic inspection
- reduce human errors in interfaces with the plant and improve supervisory functions by providing better quality information
In some cases, developers are also considering the use of fully automated facilities with remote monitoring and intervention capabilities.
REGDOC-2.5.2, Design of Reactor Facilities: Nuclear Power Plants, sets requirements and guidance for the design and safety analysis of plant structures, systems and components including instrumentation and control systems. This regulatory document also sets requirements for the human factors engineering program plan that is an essential part of the decision process for automation of systems. Although many of these instrumentation and control technologies may have been proven in other industries, their application in a nuclear environment may require additional research and development. For example, certain types of sensors may not be qualified for use in a nuclear environment.
An instrumentation and control appendix to REGDOC-2.5.2, Design of Reactor Facilities: Nuclear Power Plants, is being developed to provide requirements and guidance to practitioners in light of lessons learned from new build nuclear power projects around the world.
For the topic of "increased use of automation for plant operation and maintenance", is additional clarity needed in existing requirements and guidance related to the implementation of automation strategies for SMRs?
Specific to autonomous operation with remote monitoring and intervention, what safety and control measures could be taken to help prevent/mitigate communication loss between the SMR and the monitoring facility?
2.13 Human/machine interfaces in facility operation
For some SMR concepts, alternative approaches are being considered by technology developers for human/machine interfaces (HMIs) used for facility operation. For example:
- one operator overseeing the operation of multiple reactor units through supervisory systems
- the use of new control approaches such as state-based controlFootnote [7], which changes how plant status is communicated to the operator particularly when changing from one plant state to another (e.g., going from full power operation to a safe shutdown state)
- autonomous operation of facility systems with capability to have remote monitoring and intervention by operators either onsite or from a distance
Such new technological approaches to HMIs need to be supported by consideration of impacts on cognition that influence judgment, perception, memory and reasoning of the persons interacting with the facility systems. This means that the design process, including the development of software behind HMIs, must include an integrated human factors engineering (HFE) program. The technology developer's HFE program is key to demonstrating that the potential HMI characteristics are well understood and addressed in the design of facility systems. The HFE program also provides information to programs for training and certification of facility staff.
These, in turn, can help:
- reduce operational and maintenance errors that could lead to events
- promote timely and effective response to events at the facility under the various plant states including accident management
- improve communication of key facility information to offsite emergency response support organizations
REGDOC-2.5.2, Design of Reactor Facilities: Nuclear Power Plants, sets requirements for the HFE program plan. Other regulatory documents related to this are:
- G-276, Human Factors Engineering Program Plans
- G-278, Human Factors Verification and Validation Plans
Potential licensees will be expected to demonstrate how they meet CSA Group standard N290.12 Human factors in design for nuclear power plants, and therefore are likely to expect the same from their vendors.
Existing requirements cover most, if not all, of the design aspects required to design HMIs capable to support oversight and control of SMRs. CNSC regulatory documents for design and safety analysis articulate requirements for both design of HMIs and the HFE programs needed to show that the HMIs will be an effective contribution to facility defence in depth. Other regulatory requirements address the need to consider:
- cyber security
- operator response time to events (also known as "grace time")
- accident management
- failure of logic that could lead to a misdiagnosis or adverse operator action
- impacts on field status monitoring
- fitness for duty implications of higher level of reliance on the HMIs
The CNSC recognizes that some clarifications may be needed for specific applications of HMIs such as offsite monitoring and intervention of autonomous systems and in this specific case questions around onsite complement are likely to be posed by technology developers.
For the topic of "human/machine interfaces in facility operation", the CNSC is seeking comments from technology developers who are proposing new HMI technologies approaches/architectures for use in SMRs.
Is additional clarity needed in existing requirements and guidance for HMIs used for facility operation and maintenance? If so, what areas could benefit from additional clarity?
2.14 The impact of new technologies on human performance
Human performance may be described as the outcomes of human behaviours, functions and actions when carrying out work tasks. It includes the decisions and actions made while doing work, and the end result of such activity. Human performance can be influenced positively and negatively by various factors (e.g., training, work environment, communications, job procedures, and the physical design attributes of equipment and tools). Human factors need to be considered explicitly during design, with a view to supporting human performance in all plant phases including manufacture, construction, commissioning, operations, maintenance, refueling and decommissioning. In order to optimize human performance, it is necessary to control factors that can have a negative effect on humans doing work.
The existing regulatory documents relating to human performance include:
- REGDOC-2.2.2, Personnel Training
- REGDOC-2.2.3, Personnel Certification
- REGDOC-2.5.2, Design of Reactor Facilities: Nuclear Power Plants
- RD-204, Certification of Persons Working at Nuclear Power Plants
- P-119, Policy on Human Factors
- G-276, Human Factors Engineering Program Plans
- G-278, Human Factors Verification and Validation Plans
- G-323, Ensuring the Presence of Sufficient Qualified Staff at Class I Nuclear Facilities – Minimum Staff Complement
- RD-363, Nuclear Security Officer Medical, Physical, and Psychological Fitness
Human performance is a key contributor to the safety of nuclear facilities. As discussed throughout this paper, multiple features are being introduced into SMR concepts and designs with the intent to enhance facility performance in areas such as:
- safety
- operability and maintainability
- waste reduction
- lowering dose to workers
- advanced automation concepts
Many of these approaches are identified in this paper as being novel approaches, particularly when implemented together as part of an overall plant operational plan. For example, the greater use of automation in maintenance (i.e., prognostication tools) can impact how decisions are made for operation and maintenance.
However, even with the use of improved design measures, human performance plays a major role in two key areas:
- The proponent should have a program in place to ensure that human factors considerations are formally and systematically considered in the design of the facility through requirements that influence human performance. This applies to design of the overall concept and also to design of the constituent parts
- The licensee is expected to monitor, evaluate and reinforce effective human performance within its organization
The proposed location of SMRs (such as an isolated location) may pose unique challenges to licensees in maintaining an acceptable human performance program to manage human factors in the support of workers.
The CNSC is currently working on a separate discussion paper on human performance and will request feedback on this specific topic in the near future.
For the topic of "The impact of new technologies on human performance" is additional clarity needed in existing requirements and guidance for human performance in an SMR environment?
2.15 Financial guarantees for operational continuity
The CNSC anticipates cases where future nuclear power build projects would be funded under alternative ownership models. Examples include:
- ownership by foreign consortia
- fleets of very small SMRs operated under a factory build-own-operate model
In the second example, a number of developers are considering deployment of SMRs into remote parts of Canada where the power from the reactor would be critical infrastructure important to life.
The CNSC has the authority under the NSCA to require financial guarantees, if necessary, to mitigate business continuity conditions if the ownership model puts the licensee at risk of being inadequately funded by the owners (due to, for example, business failure). These funds would, for example, be used to pay for necessary expenses to:
- conduct key licensed activities related to safety, security and environmental protection
- maintain the facility in a specified safe state for a predetermined period of time
- decommission the facility
The Commission may require a financial guarantee if the Commission considers this necessary for the purposes of the NSCA. To that end, the CNSC has implemented financial guarantees for all major nuclear facilities and uranium mines and mills across Canada. The financial guarantees have been considered by the Commission and are included as part of the licensing basis for each applicable nuclear facility.
The scope and amount of a financial guarantee would be based on the funding necessary to ensure a safe state for the facility until such time as alternate funding can be arranged to allow operation to resume, or the facility to be decommissioned. The guarantee is generally based on a plan put forth by an applicant as part of a licence application.
Currently, the CNSC requires licensees of reactor facilities to have financial guarantees to ensure that there will be sufficient resources to safely terminate licensed activities. These are known as decommissioning financial guarantees and are discussed in regulatory document G-206, Financial Guarantees for the Decommissioning of Licensed Activities. When licensees terminate their activities, they must properly account for the safe disposal of all licensed material and equipment, and must demonstrate that all locations associated with the licence are free of radioactive contamination. Failure to properly terminate licensed activities can result in risk to the health and safety of persons and the environment. A financial guarantee does not relieve licensees from complying with regulatory requirements for termination of licensed activities, but ensures there are funds available in the unlikely event that the licensees are unable to carry out safe termination.
For the topic of "financial guarantees for operational continuity", is additional clarity needed in existing requirements and guidance related to the implementation of financial guarantees for operational continuity to ensure safe conduct of licensed activities?
Are there other financial instruments not listed in G-206 that would be useful in helping put financial guarantees in place?
2.16 Site security provisions
Developers of SMR technologies are seeking alternative approaches to security, such as security by design, in order to reduce the need for security personnel.
The nature of nuclear power, information concerning design and operation of nuclear facilities, and the activities conducted at a nuclear facility require a highly secure environment as part of the overall safety approach. This is reflected in the definition of a high-security site contained in the Nuclear Security Regulations:
"'high-security site' means a nuclear power plant or a nuclear facility where Category IFootnote [8] or II nuclear material is processed, used or stored."
Security approaches typically involve a combination of measures including engineered features, administrative measures and use of highly qualified security personnel. The CNSC is aware that SMR developers are seeking to increasingly employ design measures (i.e., security by design) to reduce the need for onsite security personnel. One of the concerns raised by proponents in a number of countries is that security requirements are not sufficiently flexible to address such a design approach.
The Nuclear Security Regulations generally permit a measure of flexibility in the use of alternative approaches while ensuring security will remain commensurate with the proposed activities. The regulations permit the application of a graded approach particularly as they apply to the security requirements for nuclear material. For example, sabotage scenarios would need to be considered taking into account all features and consider where inventory is stored and in what state the material inventory is in (e.g., fresh fuel, waste fuel, in the core and others).
Section 3 of the Nuclear Security Regulations requires the licence applicant to perform and submit a site-specific threat risk assessment, and substantiate it in a security program document and site security plan, including information around how they would meet requirements. This would also include how security by design would be performed.
- Regulatory documents for this safety and control area include:
- RD-321, Criteria for Physical Protection Systems and Devices at High-Security SitesFootnote [9]
- RD-361, Criteria for Explosive Substance Detection, X-ray Imaging, and Metal Detection Devices at High-Security SitesFootnote [10]
- REGDOC-2.12.1, High-Security Sites: Nuclear Response Force
- REGDOC-2.12.2, Site Access Security Clearance
CSA Group standard N290.07-14, Cyber security for nuclear power plants and small reactor facilities also supports the regulatory document requirements listed above.
For the topic of "site security provisions", what regulatory issues may present challenges to deployment scenarios for SMR facilities? For example:
- How could subsurface or civil structures be implemented as part of the security by design approach?
- How might security provisions differ for SMRs with a very limited onsite staff and located in a remote region? How would possibly lengthy offsite response times be addressed?
- How would security provisions be addressed for offsite monitoring/control of facilities if used?
2.17 Waste management and decommissioning
All industrial processes, including the production of nuclear energy, create waste. Of these, some are easily managed and disposed of; others may be longer lasting. The CNSC recognizes that the public considers waste management and disposal strategies to be a high profile issue.
The CNSC licenses, regulates and monitors Canada's waste management facilities to ensure they are operated safely. The CNSC imposes rigorous reporting requirements on the operators of nuclear waste management facilities, and verifies that facilities comply with established safety requirements through inspections and audits.
As a facility approaches the point of decommissioning, its licensee is required to prepare and submit a detailed decommissioning plan for CNSC approval. This plan is a refinement of the preliminary decommissioning plan, and is used to present the results of post-operational contamination surveys, hazard assessments, environmental impacts, and new technological or regulatory developments that may change the decommissioning strategy.
Regulatory approach to waste and decommissioning
In the Class I Nuclear Facilities Regulations, every licence application must include information about the waste management program to be implemented for the licensed activities and the proposed plan for decommissioning of the nuclear facility or site. This information is used to understand potential hazards and mitigate potential environmental impacts. The level of information requested increases as a facility progresses through site preparation, construction, operation and decommissioning. This information also informs the decommissioning guarantee.
One of the key principles in IAEA guidance and CNSC regulatory policy P-290, Managing Radioactive Waste, is that the licensee minimize the generation of radioactive waste.
Section 14 of RD/GD-369, Licence Application Guide: Licence to Construct a Nuclear Power Plant outlines the CNSC's expectations for information on waste management that must be included in a licence application for the proposed activities. Section 15 of the guide articulates the CNSC's expectations in a licence application for decommissioning and end-of-life aspects.
G‑219, Decommissioning Planning for Licensed Activities, provides guidance on the preparation of decommissioning plans for activities licensed by the CNSC and provides information for the basis for calculating financial guarantees discussed in G-206, Financial Guarantees for the Decommissioning of Licensed Activities.
Both the CNSC environmental assessment and the licensing process for specific projects require applicants to address the question of waste management from the onset of the project. All of the existing requirements and guidance would apply to SMR projects.
The CNSC has an optional pre-licensing vendor design review process in place prior to any licensing process being triggered. The process permits a reactor vendor to obtain regulatory feedback regarding specific aspects of the design. The focus area that deals with decommissioning in design treats the ways in which the vendor plans, via upfront design measures, to facilitate the facility decommissioning process. The review focuses on the vendors' approach to address the materials described in section 7.24 of REGDOC-2.5.2, Design for Reactor Facilities: Nuclear Power Plants. This feedback will allow a vendor to prepare to demonstrate approaches to a utility that is seeking to address this as part of the licence application process.
Waste management issues presented by small modular reactors
The range of types and sizes of SMRs means that the types and characteristics of waste may, in some cases, be different from what is being generated in existing Canadian facilities. For example:
- Low-level wastes are likely to remain similar in type (e.g., lightly contaminated tools, used clothes, cleaning supplies) and composition but the volumes generated are likely to be significantly lower due to the smaller facility size and conscious design decisions being made by developers.
- A number of SMRs propose to use of different types of reactor coolants, purification schemes and approaches to inspections and maintenance. This may mean that wastes such as used filters, ion exchange resins and even contaminated components from maintenance and in-service inspections may present different contaminant characteristics and therefore need to be managed and disposed of in alternative ways. Different fuel enrichments and types will be utilized from 4 percent enrichment for light water reactors and standard fuel, up to just below 20 percent enrichment for compact and smaller factory fuel conceptsFootnote [11]. Fuels may range from traditional elements used in today's reactors to metallic, graphite, carbide and molten salt. Processes for handling and storage will require their own methodologies to be developed, but fundamental requirements will remain the same.
- The use of alternative approaches to operate and maintain the various systems in SMRs may result in different types of hazardous wastes from those generated in current facilities. Again, the quantities of such wastes are likely to be significantly lower due to the combination of factors such as smaller facility size, conscious design decisions, and federal, provincial/territorial and municipal environmental requirements that place the responsibility for dealing with waste on the operator.
Decommissioning issues presented by small modular reactors
SMR concepts may introduce some new approaches to decommissioning. For example:
- A multiple module facility may seek to replace complete reactor modules as part of the operational strategy. Refurbishments of reactors are treated as normal waste management.
- For smaller transportable (replaceable) reactors, site decommissioning and reactor decommissioning may be performed as separate activities and even under different licences.
For the topic of "waste management and decommissioning", what are some of the key strategies for waste management, spent fuel management and decommissioning that the CNSC and licensees need to consider for various SMR deployment scenarios? For example, for companies considering a fleet of SMRs across a wide geographical area, how would waste and decommissioning be addressed?
In implementing these strategies, where are the challenges that exist in interpretation of current requirements and guidance?
2.18 Subsurface civil structures important to safety
RD/GD-369, Licence Application Guide: Licence to Construct a Nuclear Power Plant, articulates the CNSC's expectations in a licence application for civil structures.
REGDOC-2.5.2, Design for Reactor Facilities: Nuclear Power Plants, provides requirements and guidance for design of civil structures.
One of the key defining features of many proposed SMR designs is that the reactor and systems and structures important to safety and security (known in the industry as the nuclear island) will be located either partially or fully underground. As a concept, this idea is not new. It was first envisioned in the 1950s in most of the countries that first developed nuclear technologies. Examples of such past efforts exist in Canada at the Nuclear Power DemonstrationFootnote [12] power reactor in Rolphton, Ontario and the Whiteshell WR-1Footnote [13] prototype organic cooled reactor in Pinawa, Manitoba. Among the primary reasons being touted by vendors for placing a reactor building below ground are:
- added structural strength afforded by the surrounding rock
- the possibility to further reduce potential for fission product release in the event of a serious reactor accident
- a reduced threat profile against external hazards such as aircraft crashes
- easier to secure the facility from unauthorized entry
- more difficult to remove materials from the facility
- protection against the effects of most severe weather events and some potential protection even from the effects of earthquakes (flood mitigation may require more attention, depending on the site)
- use of natural radiation shielding materials (i.e., rock and soil)
For subsurface structures, understanding and mitigating the impacts of potential external hazards (such as flooding and ground motion, static and dynamic physical forces and aging management related phenomena) will be important to the safety case of the facility over its service life, which could be as long as 100 years. The body of knowledge generated for this engineering approach is also highly necessary to support submissions in a project's environmental assessment, particularly in support of subsurface groundwater.
An engineered civil structure acting as a barrier is highly important to the safety and environmental protection strategy of a nuclear facility. It is part of the defence in depth approach to prevention and mitigation of the release of radioactive and hazardous substances into the environment. Subsurface structures present engineering challenges to detecting and assessing physical degradation of materials in structures important to safety and environmental protection. Although experience with the use of underground structures exists in other industries, in many cases the technologies for aging management of civil structures have not been proven in a nuclear environment. Although normally referenced in a reactor licence, Canadian and American codes and standards for nuclear structuresFootnote [14] do not currently contain provisions to address deeply embedded structures.
For the topic of "subsurface civil structures important to safety", to complement the CNSC's investigation into ageing management of civil structures, where is SMR industry work is being performed in this area to address aging management issues in codes and standards? Of particular interest is ongoing work being done on technologies necessary to reliably demonstrate that such structures remain fit for service over the life of the facility including provisions for safe storage and decommissioning plans?
3. Fusion technologies
All nuclear reactors around the world use nuclear fission, in which a controlled chain reaction is established to split atoms of certain heavy elements (such as uranium-235) to generate heat.
Another method to generate heat is called nuclear fusion. With fusion, two or more atomic nuclei come very close. They collide at a very high speed and fuse together to form a new nucleus. Nuclear fusion is seen by many to have potential advantages over fission. It produces significantly less waste by-products and lower overall hazards. However, achieving manmade fusion has proven incredibly challenging. Research into controlled fusion, with the aim of producing fusion power for the production of electricity, has been conducted for over 60 years without success. Regardless, there continue to be numerous initiatives around the world, including within Canada, to develop fusion power technology.
Currently, should a proponent come forth with a fusion technology and seek to construct and operate it in Canada, the Class I facilities regulations would apply. The definition of a Class IA facility, as currently stated in section 1 of the regulations, is as follows:
"Class IA nuclear facility means any of the following nuclear facilities:
- a) nuclear fission or fusion reactor or subcritical nuclear assembly; and
- b) a vehicle that is equipped with a nuclear reactor"
Nuclear fusion activities are recognized to have different, but not insignificant risks to health, safety and the environment. However, the CNSC notes that many of these risks are likely to be lower in magnitude when compared to larger fission reactors. In general, hazards related to fusion development and operation may include a combination from the list below. These would need to be taken into consideration for each type of fusion equipment, and proper hazard analysis would be required based on specific design solutions:
- tritium handling (e.g., remote handling requirements)
- tritium releases (operational and accidental)
- fast neutrons (fusion releases high energy neutrons)
- other than neutrons ionizing radiation other than neutrons resulting from fusion reactions
- mobilization of activated materials; neutron activation of materials
- hydrogen explosion
- lithium and other fire hazards
- dust related to molten metal circulation (explosion, toxicity)
- erosion of the molten metal transport systems (metal to metal friction) and transport of radioactive particles in it
- active magnetic fields (effect on humans and consequences of failure)
- plasma containment failure
- chemistry of materials
- electronic control systems and external effect on them (plasma, high currents and magnetic fields)
Where future fusion-fission hybrid nuclear installations will want to breed fissile materials, within special blankets (e.g., thorium or uranium based), a risk of plasma containment and the blanket meltdown and criticality must be considered.
Regulation of any activities associated with the development and use of such technologies needs to address the hazards that may exist in the conduct of proposed activities. Many of the existing tools as well as requirements used for nuclear fission reactors will continue to be applicable, such as use of defence in depth, safety analysis and radiation protection. As a result, they would be used as a starting point in any near term proposals for fusion reactor development projects.
For the topic of "fusion technologies", what are the types and magnitudes of risks and hazards that would be posed by different fusion technologies (conventional and radiation hazards)?
With this in mind, how would the risks posed by activities involving fusion reactors differ from current nuclear fission reactors? Should fusion reactors be regulated differently than fission reactors?
4. Conclusion
Over the past several years, the CNSC has seen increased interest from potential vendors, licensees, utilities, other levels and departments of government, and other interested stakeholders in small modular reactors (SMRs). But what are the novel or unique regulatory and licensing implications presented by SMRs? If a proponent decides to deploy such a technology in Canada, what are some of the key licensing issues that need to be resolved to meet Canadian requirements?
This discussion paper has attempted to illustrate that Canada's nuclear regulatory framework and approach to regulation has the flexibility to allow a licence applicant to propose innovative approaches to address safety and environmental protection without compromising safety and environmental protection.
The paper has also sought to establish that SMRs, with the current technology-neutral safety and environmental protection requirements for nuclear power plants, would be able to be considered for CNSC licensing, assuming the applicant can demonstrate that the SMRs are safe. Furthermore, the CNSC would provide for effective SMR regulatory oversight to prevent an unreasonable risk to the environment, to the health and safety of people and to national security, associated with the development, production, possession or use of SMR technologies.
However, even with a flexible regulatory approach, some innovative features may present challenges in both interpretation and application of requirements. This paper has attempted to highlight a number of key areas where potential challenges could exist. In some cases, the CNSC can confirm that the existing requirements remain valid and useful. In a number of other areas, the implications of the innovative approaches need to be examined to a greater degree to confirm whether additional requirements or guidance are needed to further support those already existing.
This discussion paper has presented an overview of a number of potential issues that the CNSC has identified. These issues have been described at a high level along with a short description of specific items to be addressed in future work. In other cases, the paper presented how the CNSC plans to address the issues under its current regulatory framework.
The CNSC is seeking to hear from all interested stakeholders on the topics addressed in this paper as well as any others they feel are relevant to the discussion on SMRs.
5. How to participate
Please submit your comments or feedback to:
Canadian Nuclear Safety Commission
P.O. Box 1046, Station B
280 Slater Street
Ottawa, Ontario K1P 5S9
Fax: 613-995-5086
Email: consultation@cnsc-ccsn.gc.ca
Please indicate clearly which discussion paper you are commenting on.
Appendix A: Overview of Design Concepts for Small Modular Reactors
There are many vendors throughout the world with small modular reactor (SMR) designs at varying levels of completion. An extensive but incomplete list of reactor technologies, including SMRs, is available from the IAEA Advanced Reactors Information System (ARIS) database.
The IAEA has a free publication, Advances in Small Modular Reactor Technology Developments, which focuses on only SMR technical descriptions.
Figure 1 lists all SMR vendors who have requested regulatory information from the CNSC.
Conventional grid small modular reactors | |||
---|---|---|---|
Country of origin | Vendor name |
Reactor name (Per-unit output) |
Reactor type |
USA | NuScale Power | NuScale Module (45 MWe) | Integrated light-water reactor (LWR) |
Korea | KAERI | SMART (100 MWe) | Integrated LWR |
China | CNNC | ACP-100 (100 MWe) | Semi-integrated LWR |
USA | Generation mPower/B&W USA | mPower (200 MWe) | Integrated LWR |
USA | Westinghouse | Westinghouse SMR (225 MWe) | Integrated LWR |
Japan | Hitachi-GE | DMS (300 MWe) | Boiling water reactor |
USA | Advanced reactor concepts | ARC-100 (100 MWe) | Liquid sodium |
USA | Holtec | Holtec Module (130 MWe) | Semi-integrated LWR |
Canada/USA | Terrestrial Energy | IMSR 300 and 600 (100 MWe and 200 MWe) | Molten salt reactor |
Canada | Northern Nuclear Industries | LEADIR-PS100 (39 MWe) | Molten lead |
Canada/USA | Elysium Industries | Unnamed (size not yet determined) | Molten salt reactor |
USA | Transatomic power |
Unnamed (size not yet determined) |
Molten salt reactor |
USA | X-Energy | Xe-100 (50 MWe) | High-temperature gas |
Edge-of-grid / Off-grid small modular reactors | |||
---|---|---|---|
Country of origin | Vendor name | Reactor name / per unit output | Reactor type |
Canada/ USA | Terrestrial Energy | IMSR 60 (28 MWe) | Molten salt reactor |
Canada/ USA | StarCore Nuclear | StarCore Module (10 MWe) Transportable | High-temperature gas TRISO prismatic |
Canada | LeadCold Nuclear | SEALER (3 MWe) Transportable | Molten lead – uranium nitride |
USA | Gen4Energy | Gen4 Module (25 MWe) Transportable | Molten lead bismuth |
Appendix B: Additional Background Information
This appendix gives background information to further elaborate on the fundamentals/rationale for regulation for the topics discussed in section 2 of this paper. The main audience for this information is persons who are less familiar with the Canadian approach to regulation and are seeking to understand why these areas are important to safety. This information does not establish any new regulatory policy and has been compiled from diverse information from throughout the CNSC's existing regulatory framework.
B.1 Background
B.1.1 Understanding what an SMR is and what it represents
The meaning of the term SMR varies from country to country. Even the acronym is not universally agreed upon, having been interpreted as:
- small modular reactor
- small and medium reactor
- small and medium modular reactor
SMR designs can conceptually be placed into one of four categoriesFootnote [15]:
- Ready to build: These are similar in concept to, but smaller than current water-cooled reactors. They may use an integral design in which reactor vessel, control rod drives, steam generators, and pressurizers are all located inside a single module. They are described as ready to build because they claim to be based on existing proven technologies and concepts.
- Second time around designs: These are different from light-water reactors. They were explored from the 1950s to 1970s, but were abandoned in favour of light-water designs. These include reactors cooled by gases such as helium, liquid metals such as sodium or lead, or molten salts.
- Nuclear Waste Disposal Reactors: These are mostly so-called "fast-reactor" concepts that can transmute isotopes from existing spent fuel into usable fuel or more short-lived waste products. This would require processing of spent fuel from existing reactors to package the fuel for use in this type of reactor. Past examples of these designs have operated. Larger fast reactors would not be SMRs but rather full-scale power plants.
- Lifetime fuelled reactors: Also known in the popular media as "nuclear batteries", these reactors would be very small (3 to approx.35 MWe), fuelled once before deployment and either decommissioned when depleted or refurbished and refuelled off the customer site at a service facility. The International Atomic Energy Agency (IAEA) calls these concepts transportable nuclear power plants (TNPPs).
What is clear is that SMRs will be nuclear fission-based reactors, although smaller than current traditional designs with alternative technologies used to achieve safety and economy. They are being designed, for the most part, to serve different power markets: i.e., to generate electrical power or process heat in regions where an NPP would be too large.
Proponents also claim other positive attributes for SMRs. Many new large reactor designs also have some of these attributes, but the important consideration is that SMR designers are applying more of these attributes and to a greater degree. The following are the primary features highlighted:
- Modularity: Many proposed SMR designs would be made of engineered modules manufactured in a serial manner and in a controlled factory environment. The premise is that factory manufacturing results in higher quality construction, lower manufacturing times and economies of scale. These engineered modules would be delivered from production factories to be assembled on the plant site. The assumption is that construction time would be significantly reduced. It is also claimed that some of the commissioning work could be done during manufacturing, reducing the onsite time to bring the plant to commercial operation. This concept has been proven in the shipbuilding and aerospace industries. Some modern large reactor designs are also using this approach.
- Simplified design features: SMR designs are generally being developed to reduce the numbers of components and systems and to use so-called passive and inherent safety features as part of their safety approach. Modern large designs are also using this approach. Simplified design features may mean less use of pumps, valves and pipes. Some reactor designs may be self-contained modules containing the core, reactivity mechanisms, steam generators, pressurizers, and circulation pumps in a single vessel. Reactor designers claim that fewer components allows for higher reliability.
- Passive features: These are safety features that do not require operator actions or electronic feedback in order to, for example, shut down the reactor safely in the event of a particular type of emergency (such as overheating resulting from a loss of coolant or loss of coolant flow). These features rely on the engineering of components such that they behave according to known laws of physics. An example of a passive feature typically used in SMRs is an emergency cooling water tank that drains by gravity into the reactor vessel to keep the fuel covered for a long period of time, in the unlikely case of a loss of primary coolant accident, to delay fuel melt.
-
Inherent safety features: These are defined by the actual characteristics of the reactor
itself; for example:
- a reactor core with a small core inventory or shape that may significantly reduce or eliminate fuel melt because of its natural ability to shed heat without external assistance.
- a reactor's fuel and moderator arrangement that may result in a situation where any disturbance in the reactor (e.g., boiling in the coolant, or fuel temperature increase) will result in a natural loss of reactivity (negative coefficient of reactivity)
B.1.2 If all of these new concepts are improvements then what are the regulatory issues?
Technologies and features must be demonstrably safe; i.e., sufficient scientific and engineering evidence must exist to support that a new or novel feature will do what the designer says it will do with very high certainty.
Most SMR concepts being proposed, although based on past technological work and operating experience from past and existing plants, employ a number of novel approaches simultaneously. These approaches impact the certainty of how a plant will perform under not only normal operation but also under accident conditions where predictability and certainty of system operation is paramount to safety. Uncertainties in a design are always a focus of regulatory discussions, as understanding and minimizing uncertainties leads to reduced risk and improved safety. Uncertainties need to be well understood. They can be addressed by implementing other strategies to reduce potential risk such as:
- designing a component to be more robust than it may need to be
- adding, for example, redundancy to improve safety if a single component fails
This is a concept used in many industrial sectors. Evidence is normally gathered by the designer during the design of a new concept via research and development (R&D) activities including experiments and computer modelling simulations. In some cases, experience about a concept may exist in other industries. Where this is the case, the designer must compare how the concept is adaptable for nuclear application and perform R&D to address any gaps.
Application of multiple novel approaches to achieve design targets can result in potential new regulatory issues such as:
- analyzing complex interactions between new features under varying facility operating conditions
- developing new maintenance approaches
- understanding how new materials will perform over the long term (for example, understanding corrosion and erosion of metals)
- uncertainties in whether, for example, passive features will operate as predicted under all plant conditions
Reactor size and type are not key regulatory issues in Canada; therefore, this discussion paper focuses on the implications of certain novelties being introduced in these new technologies
B.1.3 Where SMRs might be deployed
Note: The information in this section has been supplied for context only. It does not provide or imply any opinion or attempt at promotion on the part of the CNSC on energy or nuclear policy.
Canada's geography, varying population distribution and resource-based economy mean that different types of power generation infrastructure are needed in different parts of the country.
Most of Canada's population centres are based in southern parts of the 10 lower provinces and are served by interconnected provincial grids (see the Canadian Electricity Association's electricity map for an interactive diagram). Each provincial grid evolved according to the corresponding provincial energy policy, population distribution and industrial capacities – with some grids being served by many smaller generating facilities and others being served primarily by large generating facilities.
Where provinces are served by smaller grids, the introduction of a large electrical generating facility has the potential to introduce grid stability problems because of a large generating source in one location. In addition to this, upgrades to grid infrastructure would be necessary to accommodate the power output of a large facility. A number of SMR vendors are developing and implementing concepts in the 50 to 300 electrical MW (MWe) per generating unit range to fit an existing grid without requiring major grid modifications. The predominant use for SMRs in this instance would be for electrical power generation with the generators replacing older fossil-fired facilities. Some vendors are also proposing the use of certain SMR technologies to supply bulk electricity or process steam for large mining projects
In northern regions of Canada, the energy picture is completely different and a number of reactor vendors are working on smaller power solutions for northern applications. In these regions, electrical power is either "edge-of-grid" or "off-grid".
Edge-of-grid regions are generally served by very long but simple transmission lines connected to the southern transmission systems. These northern systems cover large linear distances but have little or no power transmission redundancy. As a result, these systems are vulnerable to more frequent and lengthy power interruptions. If there is a need to supply power to a region that is not served by the existing feeder line (e.g., to provide power to a new mine), a decision needs to be made on whether to expand the grid into that region or, alternatively, to service the region with off-grid or remote generation sources. Traditionally, due to the need for highly reliable and non-variable sources, fossil-fired (diesel, propane, liquefied natural gas) units are generally used.
In the far north of Canada – where the small permanent population is located in small towns or resource based projects (mines) spaced hundreds of kilometres apart – there are no interconnecting grids and all power must be generated locally for local consumption. Generation requirements range from as low as 500 kWe to as high as 5 MWe for communities and up to 45 MWe (electricity plus using the process heat) for a large mining project.
For edge-of-grid or even off-grid applications, the CNSC is aware that several vendors are considering SMR concepts in the 3 to 35 MWe (per unit) range, depending on customers' power needs. These are being considered to supplement an existing northern or remote grid-system or as an off-grid source. A unique feature being contemplated for some of these design concepts is the lack of onsite refuelling. Some vendors are proposing that these designs be factory-fuelled and then shipped as sealed units to a site for installation and operation. Once depleted, the intent is to remove them from the site and either return the units to the factory-of-origin for refurbishment/decommissioning, or place them into a used-fuel repository. These sealed and transportable units, in the language of the International Atomic Energy Agency, are called transportable nuclear power plants – but shall be referred to as transportable reactors in this paper.
All of these different scenarios present various policy and technical issues that industry, governments and regulators need to address. These issues are identified and discussed later in this paper.
B.2 Additional information about information to support a safety case
Technical information such as that obtained from R&D supports various aspects of an application for a licence, such as the credibility of a safety claim, effectiveness of a safety approach or the informing of long-term considerations in the conduct of licensed activities. The importance of this information is reinforced in the General Nuclear Safety and Control Regulations, in, for example, section 3(1)(i), which requires an application for a licence to contain "a description and the results of any test, analysis or calculation performed to substantiate the information included in the application".
Such information is necessary to demonstrate performance or that adequate safety margins exist. It also helps to understand:
- performance characteristics of features (both separately and in combination with others) in nuclear specific environments
- uncertainties that may arise with the use of features and what those uncertainties mean in the overall demonstration of safety
- applicability and sufficiency of past R&D efforts and external operating experience to the current case (What gaps need to be address between the current case and past work?)
- areas where existing or new simulation software needs to be improved to ensure the best possible understanding of future reactor performance
Because the application for a licence becomes part of a licensing basisFootnote [16], which is encompassed by the licence, technical information (such as from an ongoing R&D program) and its impacts on the safety claims are expected to be revisited regularly by licensees as methodologies change, assumptions change or other new information emerges, such as that obtained from operational experience.
A CNSC technical assessment of a licence application examines each applicable safety and control area to confirm that the applicant has demonstrated that the information is pertinent, credible and sufficient, and that the applicant is drawing from a living quality-assured R&D program. For example, one factor that CNSC looks for in R&D activities is how the use of computer simulations for design is balanced with the use of physical models and experiments to develop the best possible understanding of physical phenomena. The CNSC expects licensees to review their R&D information and be aware of any gaps that may exist and how they will be addressed in a timely manner. This activity continues over the facility's lifecycle.
B.2.1 Use of computer codes to support research and development activities and safety claims
All computer codes, including simulation technologies, intended for use in safety analyses and R&D activities are expected to be verified and validated by using experimental data. This is to provide assurance that all of the various safety features in the defence-in-depth framework can demonstrate safe facility operation. Features include but are not limited to:
- fuel and physics performance
- safety systems and systems that support safety
- control architecture
- human factors and performance (for normal operation as well as for plant events)
It is the proponent's responsibility to ensure that the computer programs used in R&D activities and design and safety analyses of nuclear power plants and research reactors and the output of these programs are reliable and adequate for their intended applications. This will allow a high degree of confidence may be placed in both the programs and the results.
SMR designs are exploring alternative approaches to meeting safety requirements, such as the use of passive and inherent features. The use of such alternative approaches can introduce uncertainties to safety analysis, and need to be addressed with suitable experimental evidence to support the computer codes and simulations used to analyze operational and accident sequences. For example, the following areas are influenced by the uncertainties presented by alternative approaches:
- core neutronics
- fuel performance
- thermal hydraulics
- severe accidents
- structural analysis
- dispersion of released effluents
When considering the use of existing proven industry computer codes, it is important to understand and disposition the technological differences that an SMR design presents and how this may impact the validity and proven-ness of those codes.
CSA N286.7, Quality assurance of analytical, scientific, and design computer programs for nuclear power plants, further reinforces quality assurance requirements that will need to be addressed.
B.2.2 Use of research and development information from outside the nuclear industry
The CNSC recognizes that, in some cases there is a wealth of information available on technical approaches from other industry sectors. This information may be leveraged to support safety claims, but supporting information must also address any specific considerations of the proposed activity that may influence the R&D results. For example, materials exposed to the environment inside a nuclear reactor must be able to remain fit for service in that environment for the reactor's service life. This means that additional analysis or R&D activities may be necessary to complete already existing information.
B.3 Current licensing practice for multiple reactor facilities on a single site
Current practice for the existing fleet of multiple unitFootnote [17] nuclear power facilities in Canada has shown that a single licence enveloping all activities for the facilities on the site can be done efficiently and in consideration of:
- technical / configuration differences between units
- units of different vintage (age differences)
- units in a station that are in various lifecycle stages, for example, units operating, units in refurbishment and units in safe storage state awaiting decommissioning.
Figure 2 shows an example of this practice for the Bruce Nuclear Generating Station site, where both Bruce A and B (four units each) are licensed under a single site licence.
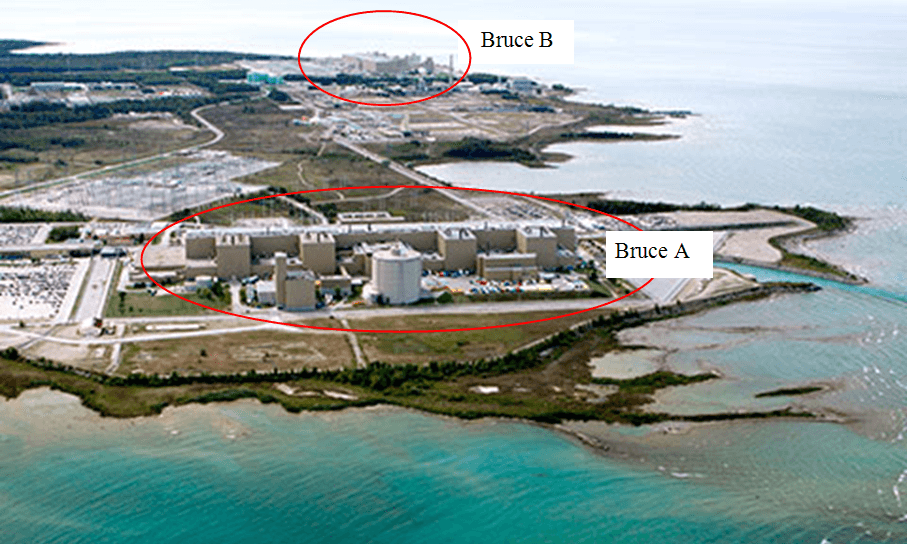
The number and nature of licences is proposed by the applicant and ultimately decided on by the Commission during the licensing process.
Operating experience with single licences for multiple-unit facilities has shown that licensees needs to consider how they will manage the differences between units as described above, in all of their programs for operating and maintaining the facility as a whole. This would include, for example, an aging management program for "common services" features that are shared between modules – including civil structures, common electrical systems and compressed air systems. This will be particularly important for cases such as:
- multiple-module SMRs where a utility proposes to put only a few modules into service at the onset, with an option to install and operate more units in the future
- spent modules that may be removed and replaced with newer modules, which could differ technically from the original unit
For a proposal for a multiple- module licence to construct or operate a facility, it important for the applicant to consider the facility's ultimate total capacity over its life and the timelines for deploying the modules. This will play a role in, for example the environmental assessment as well as the safety analyses that will support the facility's safety case. In the licence application, the CNSC expects the applicant's programs and processes to describe how multiple-unit activities will be managed under all safety and control areas. For example:
- configuration management – addressing differences between units
- human performance – personnel training and preventing errors such as performing maintenance on the wrong unit
B.4 Additional information on licensing considerations for new demonstration reactors
A demonstration reactor facility can be thought of as a full-scale and, for the most part, fully functional integrated facilityFootnote [18] with additional features built in. This permits collection of operational experience to further address technology uncertainties associated with novel approaches.
A demonstration facility could be a first-of-a-kind (FOAK) commercial facility projected to operate over a full lifecycle. Alternatively, dependent on the licensees' objectives, the demonstration program may see earlier removal from service and alternate decommissioning strategies. This must be factored into the overall licensing program, but the licensing process ultimately remains the same as for a commercial facility.
Information from the demonstration program would be used to support future licensing for FOAK / nth-of-a-kind new build projects. Information gathering by the licensee may include areas such as:
- understanding nuclear core performance under different operating conditions
- demonstration of operating concept (e.g., human machine interface validation)
- demonstration of deep-load following capability
- new materials and chemistry environments (understanding aging mechanisms)
- final stage of new fuel qualification testing
- testing alternative product streams the facility may produce (e.g., hydrogen)
Typically, demonstration facilities integrate together prototypical systems from independent engineering efforts. As a result, integrated testing and operational evolutions are performed in the demonstration facility to gather additional information on overall behaviours and performance of the complete design under real nuclear conditions.
A number of technology developers have indicated that, as part of their development plan, they will be seeking to propose the construction and operation of a demonstration facility before finalizing their design for an FOAK plant to be available to potential customers. Their stated rationale for doing this is:
- To complete R&D activities necessary to support licensing of future projects (i.e., address uncertainties associated with various new technological approaches)
- To demonstrate to stakeholders that their technology can achieve its design intent
By their nature, demonstration facilities can present additional risks as a result of the uncertainties being resolved through testing under realistic conditions. Additional safety features and increased use of conservative designFootnote [19] are used under these conditions to address potential risks. The licensing process is designed to confirm that these risks are being addressed in all safety and control areas to ensure that the activities conducted using the demonstration facilities are done so safely.
A licence application for a demonstration facility will differ in the type of evidence and operating experience available to support the safety case. For example, there may not be specific standards in place to support an engineering approach for a technology. In addition, the demonstration facility may be part of the developer's overall strategy to complete an R&D program. This means that information supporting the application must be of high quality and describe in sufficient detail how proposed approaches in lieu of evidence and operating experience will ensure safety. This information would be used as part of a regulatory discussion on use of informed engineering judgement by the applicant.
Successful approaches used around the world have included use of industrial codes and standards coupled with:
- experimental information
- supplementary safety analyses (including analyses of uncertainties)
- conservative approaches to design
Experience has shown that demonstration facility licensees generally plan for additional steps in construction, commissioning and approach to operation necessary to achieve the overall objectives of the demonstration activities. This is done to permit the performance of inspections, tests and analyses to address uncertainties as part of supporting information needed to proceed to the next phase of the project. This data also supports the technical basis for the facility's safe operating envelope. These plans are encompassed into the licensing basis by the CNSC, and inform the need for any additional licence conditions, licence hold points and compliance verification criteria.
Since licensing is focused on the proposed activities, irrespective of the technology used, the licensing process for a demonstration facility is the same as for any other reactor facility. This process is described in REGDOC 3.5.1, Licensing Process for Class I Nuclear Facilities and Uranium Mines and Mills.
B.4.1 Prototypes and test facilities
Prototypes and test facilities generally differ from demonstration facilities in the following ways:
- smaller-scale / partial-function systems designed with specific data collection missions in mind
- more limited life span
- not designed for long-term commercial use
In these cases, uncertainties are generally higher than for demonstration facilities. However, applicants would generally use the same engineering approaches to address risk and demonstrate that activities using these facilities will meet requirements.
The licensing process for activities using prototypes and test facilities will depend on the nature and extent of the activities proposed. The CNSC encourages early engagement and discussions with its staff to understand the appropriate licensing path and applicable requirements for those activities.
B.5 Background on licensing process and environmental assessments for fleets of SMRs
For edge-of-grid or even off-grid applications in remote parts of Canada, the CNSC is aware that several vendors are considering SMR concepts in the 3 to 35 MWe (per unit) range, dependent on customers' power needs. They are being considered by vendors as either supplementary to an existing northern grid-system or as an off-grid source. This may set the stage for fleet ownership of small, but similar – if not identical – facilities across a wide geographical area.
The CNSC's licensing experience has involved multiple sites run under a single company; however, the practice has been to have activities for each site encompassed under a site-specific licence. To date, no proposals have been made to have multiple sites encompassed under a single licence.
The construction, operation and decommissioning of any new nuclear fission or fusion reactor is considered a designated project as defined in section 2 of the Canadian Environmental Assessment Act (CEAA 2012) and outlined in the associated Regulations Designating Physical ActivitiesFootnote [20]. For designated projects described in the CEAA 2012 that are regulated under the Nuclear Safety and Control Act (NSCA), the CNSC ensures that CEAA 2012 requirements are met. It is important to note that a proposed SMR project may also require an EA by another provincial or territorial jurisdiction. Furthermore, in many parts of northern Canada (e.g., Yukon, Northwest Territories, Nunavut, parts of Quebec and parts of Newfoundland and Labrador), EA processes established under land claim agreements apply, and the CEAA 2012 does not. In these areas, CNSC acts as a technical advisor throughout the EA process and has no EA decision-making role. The Commission retains decision-making on licensing matters under the NSCA. To the extent possible, where multiple jurisdictions are involved, these processes are harmonized to reduce duplication and to provide regulatory efficiency.
CNSC's licensing process provides for significant flexibility. The EA and various licence applications can be reviewed in parallel, or in series. As discussed, under the NSCA, the Commission may also consider applications for combinations of activities; for example, a licence to prepare site and construct or licence to construct and operate, as long as the proponent addresses all requirements associated with the proposed activities. The public and Aboriginal groups are involved, as appropriate, throughout the process, including participation at public hearings. Figure 3 depicts the process for addressing each licensing phase.
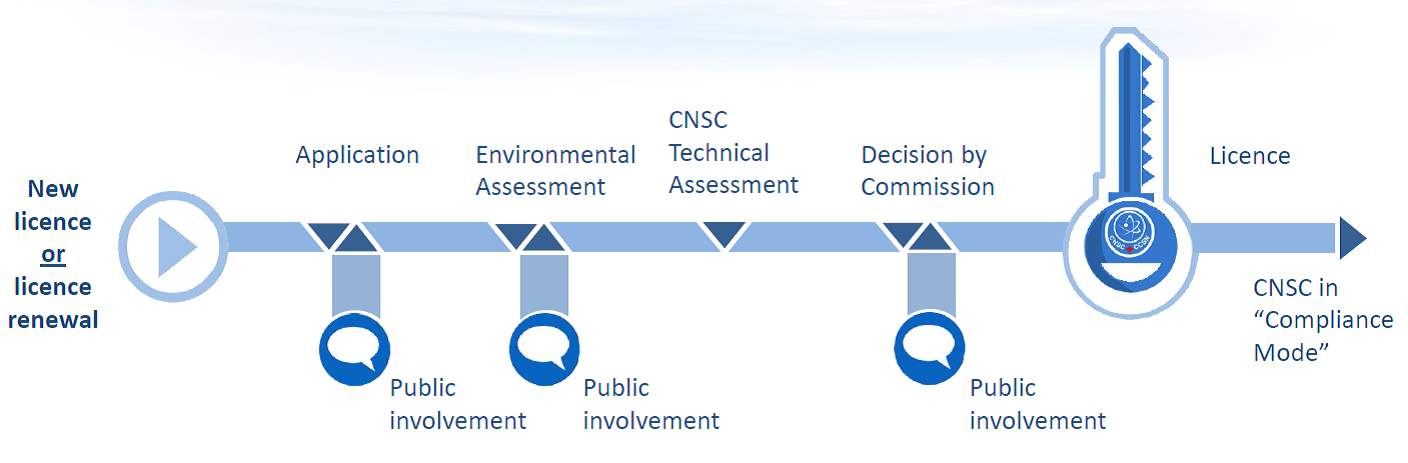
Long description
The diagram depicts the process for each licensing phase of a facility’s lifecycle from submission of an application through environmental assessment, technical assessment by CNSC staff and decision making by the Commission, to issuance of the licence and onset of the compliance program. Public involvement is included in all key steps of the process.
As part of the application process for a licence to prepare a site, a comprehensive site evaluation and the EA activities for the facility's projected life are identified and described by the proponent and assessed by the CNSC. Together, the site evaluation data and outcomes of the CEAA 2012 EA must demonstrate that:
- the site is suitable for the construction, operation and later decommissioning of a facilityFootnote [21]
- the proposed project is not likely to result in significant adverse environmental effects, taking into consideration the implementation of mitigation measures
It is important to note that a proposed SMR project may also require an EA by another provincial or territorial jurisdiction. In many parts of Northern Canada (e.g., Yukon, Northwest Territories, Nunavut), EA processes established under federal land claim agreements apply, and the CEAA 2012 does not. In these areas, CNSC acts as a technical advisor throughout the EA process and has no EA decision-making role. The Commission retains decision-making on licensing matters under the NSCA. To the extent possible, where multiple jurisdictions are involved, these processes are harmonized to reduce duplication and to provide regulatory efficiency.
B.6 Additional information on licensee management system considerations for projects involving small modular reactors
B.6.1 Identification of the licensee
Although the owner and financiers of the facility may be separate business entities from the licensee, it is the licensee's organization that must demonstrate to the Commission that it:
- is qualified to carry on the activity that the licence will authorize the licensee to carry on
- will, in carrying on that activity, make adequate provision for the protection of the environment, the health and safety of persons and the maintenance of national security and measures required to implement international obligations to which Canada has agreed
This means that the licensee must:
- be able to demonstrate sufficient knowledge of the safety case for all licensed activities
- be in control of all licensed activities being conducted
- understand the hazards associated with activities and how to control them
-
have sufficient competent resources within its organization to adequately oversee any work it commissions
externally; this capability will include technical, operational and managerial elements and includes:
- specifying requirements,
- supervision of contractors' work
- technically reviewing the output of contractors before, during and after implementation
For example, when considering the operation of a facility, precedent in Canada is that the operator is the licensee. This is consistent with IAEA fundamental safety principle #1 which statesFootnote [22]: The prime responsibility for safety must rest with the person or organization responsible for facilities and activities that give rise to radiation risks."
However, this does not discount from using qualified contractors to perform licensed activities under its control and oversight. The licensee is expected to demonstrate, in its management system, how they will discharge sole accountability and responsibility for ensuring safety, irrespective of its business and organizational arrangements in accordance with Canadian Standards Association CSA N-286-12, Management System Requirements for Nuclear Facilities.
A particularly important consideration for the safety of the licensee's organization is that there are no parent or owner business objectives that might impede the licensee's ability to conduct its activities safely. All those with responsibilities for safety should have authority and access to resources to discharge those responsibilities effectively. This needs to be reflected in the governance of the licensee's organization and associated management system. Where complex financing arrangements fund the licensee's activities throughout the facility's lifecycle, this is key.
The licensee's management system interfaces with parent companies, and vendors need to ensure that the licensee has appropriate access to design information and intellectual property that are not directly under its control.
Procurement of SMR modules (long-lead items)
A primary SMR feature will be the extensive use of modular manufacturing and construction approaches. Many large NPP designs are following a similar approach, but SMR vendors are seeking to move more aggressively to serial manufacturing of modules. These contain all structures, systems and components necessary for the modules to serve their respective functions in the future site facility. This approach is likely to include manufacture of complete reactor units, some to be installed and fueled onsite, and others that may have fuel installed at the factory before being sealed and delivered to sites.
For such modules, which would be "long-lead" components, the applicant needs to be aware of the need to demonstrate in its licence application that the conduct of the design and the physical design meet CNSC regulatory requirements. This is a particularly important consideration when the SMR or module design is undertaken in under one or multiple jurisdictions outside Canada, and is targeted for a non-Canadian or multinational market (these other markets and jurisdictions may not have the same regulatory requirements as Canada).
The CNSC and existing Canadian licensees already have extensive experience with long-lead components. Although SMR modules have the potential to be more complex than, for example, steam generators, the requirements and guidance governing procurement and supply chain arrangements currently in CSA N286 apply.
Construction and commissioning of factory-fueled and sealed transportable reactors
A factory-fueled and sealed transportable reactor module represents a special case long-lead item; unlike other typical plant components, the reactor module interior would be inaccessible to the site licensee. This provides further safeguards reassurances by preventing the possible diversion of fissile materials, but presents challenges for activities such as in-service inspections.Footnote [23] Some vendors are proposing that a vendor/assembler factory may consider low-power nuclear testing of the reactor module under the factory's operating licence before the module leaves the factory. The intent would be to reduce the likelihood of factory issues appearing at the site and to reduce the site's construction/commissioning times. All regulated activities occurring at the factory of origin would be conducted under the factory's licence. Figure 4 depicts a simplified diagram of the relationship between the factory of origin and the destination site.
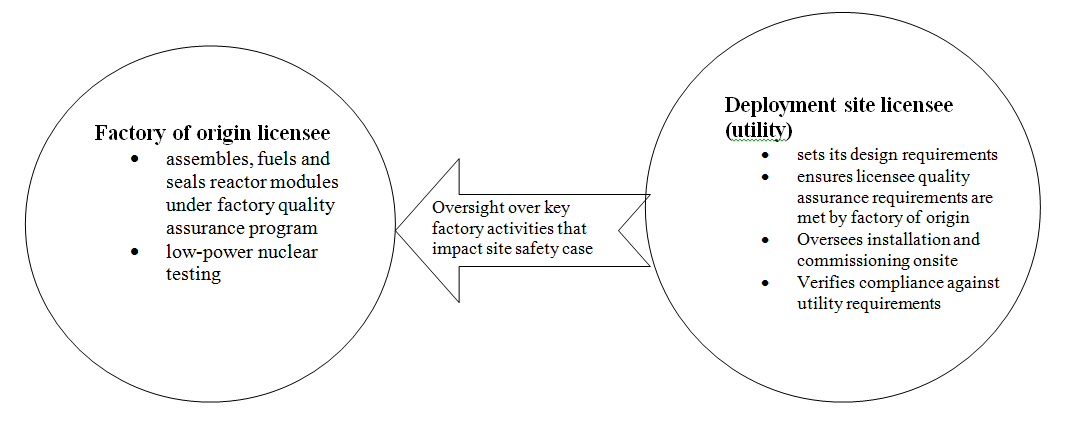
Long description
The diagram depicts the relationship that will exist between:
-
the licensee of the factory of origin (circle on the left) that manufactures and tests the factory
fuelled and sealed reactor modules (including some low-power testing)
and, - the licensee (generally a utility) at the site (circle on the right), where the sealed reactor module will be installed and operated for a period of time.
The licensee at the site is expected to have full oversight (under its licence) of any manufacturing, fueling and testing of the reactor module at the factory of origin that impacts the site licensee’s safety case. It does this by:
- setting design requirements the factory must meet
- ensuring the factory meets the site licensee’s quality assurance requirements
- overseeing installation and commissioning on its own site
- verifying compliance against the utility’s requirements
Because the partially commissioned module will be installed and placed into service at the power plant, the power plant licensee is responsible for ensuring that the module meets Canadian regulatory requirements. This means that the CNSC will expect the power plant licensee to have the management system in place to accomplish this. The licensee will be expected to show how they have developed confidence in the module's assembly, preparation and testing at the factory of origin, through factory inspections and other compliance verification activities.
Research and development information
R&D activities support not only the technology design process, but also the ongoing demonstration of safety as the facility is constructed, operated and eventually decommissioned. R&D plays a major role in the licensing process.
Regardless of who performs the R&D work, (vendors, independent laboratories, private companies etc.) SMR facility licensees in Canada need to have sufficient access to – and an understanding of – the information being used to support the adequacy of the design of their specific nuclear facilities.
B.6.2 Background information on the minimum complement in SMR facilities
One aspect of ensuring the presence of a sufficient number of qualified workers is defining the minimum number of workers with specific qualifications who will be available to the nuclear facility at all times, known as the minimum staff complement. The number and qualifications of workers in the minimum staff complement should be adequate to successfully respond to all credible events, including the most resource-intensive conditions for any facility state. This is discussed in detail in CNSC Regulatory Guide G-323, Ensuring the Presence of Sufficient Qualified Staff at Class 1 Nuclear Facilities – Minimum Staff Complement.
A minimum staff complement for a traditional NPP includes:
- certified staff (e.g. operators, health physics)
- fuel handling operators when fueling
- chemical, mechanical and electrical maintainers
- emergency response personnel
- store personnel
However, a decision not to include any of the above positions is expected to be justified by the licensee in the management system, the facility design through both the human factors engineering program and the process for establishing operational limits and conditions for the facility. This is articulated in both licence application guides and regulatory documents pertaining to design requirements.
Certification of persons
Section 21(1) of the Nuclear Safety and Control Act grants powers to the Commission to:
"(i) certify and decertify persons referred to in paragraph 44(1)(k) as qualified to carry out their duties under this Act or the duties of their employment, as the case may be"
To establish and maintain a high level of competence, appropriate certification training and qualification processes need to be in place within a managed program. The CNSC has therefore articulated requirements in RD-204, Certification of Persons Working at Nuclear Power Plants about the qualifications and the training, examination, certification and decertification of designated persons employed where a nuclear substance or prescribed equipment is produced, used, possessed, packaged, transported, stored or disposed of.
B.7 Background information on safeguards verification
The IAEA has an important independent verification role, aimed at assuring the international community that nuclear material, facilities and other items subject to safeguards are used only for peaceful purposes.
Canada has entered into safeguards agreements with the IAEA pursuant to its obligations under the Treaty on the Non-Proliferation of Nuclear Weapons (INFCIRC/140):
- Agreement Between the Government of Canada and the International Atomic Energy Agency for the Application of Safeguards in Connection with the Treaty on the Non-Proliferation of Nuclear Weapons
- Protocol Additional to the Agreement between Canada and the International Atomic Energy Agency for the Application of Safeguards in Connection with the Treaty on the Non-Proliferation of Nuclear Weapons.
The objective of these two agreements is for the IAEA to provide assurance on an annual basis to Canada and to the international community that all nuclear materials in the country are being used peacefully.
Every nuclear reactor facility type, whether a research reactor, SMR, or full-scale nuclear power plant, must have a safeguards program in place to cover the following specific areas:
- nuclear material accountancy and control (taking into account changes to fuel composition over time) for initial fuel arrival onsite through to spent fuel management
- access and assistance to the IAEA for verification inspections
- operational and design information
- safeguards equipment, containment and surveillance
The safeguards measures applied are based on the design and operation of the facilities. The CNSC is anticipating that SMR designers will provide design and features to the IAEA and CNSC at the early phase so that early consultation can be made with both IAEA and CNSC to incorporate with safeguards implementation requirements in its design and construction.
B.8 Additional information about deterministic and probabilistic safety analyses
Safety analysis activities general fall into two categories: deterministic safety analysis (DSA) and probabilistic safety analysis (PSA):
-
Deterministic safety analysis:
DSA predicts the facility's response to a range of events based on the current state of the facility as well as operator actions. This analysis addresses a range of scenarios for which the acceptance criteria must be met. It is another tool for early identification and mitigation of potential risks.
A DSA of a reactor facility's responses to an event is performed by an applicant/licensee using predetermined rules and assumptions (such as those concerning the initial facility operational state, availability and performance of the facility systems and operator actions). DSA can use either conservative or best-estimate methods. CNSC experts review licensee DSA as part of verification and compliance activities.
-
Probabilistic safety analysis:
PSA is a comprehensive and integrated assessment of the safety of a reactor facility. The safety assessment considers the probability, progression and consequences of equipment failures or transient conditions to derive numerical estimates that provide a consistent measure of the safety of reactor facility, as follows:
A level 1 PSA identifies and quantifies the sequences of events that may lead to the loss of core structural integrity and massive fuel failures.
A level 2 PSA starts from the level 1 results, analyses the containment behaviour, evaluates the radionuclides released from the failed fuel, and quantifies the releases to the environment. A level 3 PSA starts from the level 2 results, and analyses the distribution of radionuclides in the environment and evaluates the resulting effect on public health.
To demonstrate achievement of the safety objectives, a comprehensive hazard analysis, a deterministic safety analysis, and a probabilistic safety assessment are traditionally carried out. These analyses identify all sources of exposure, in order to evaluate potential radiation doses to workers at the plant and to the public, and to evaluate potential effects on the environment. Safety analyses are analytical evaluations to demonstrate how safety requirements and expectations, such as ensuring the dose acceptance criteria, and the integrity of barriers against releases of radioactive material and various other acceptance criteria, are met for all initiating events that could occur over a broad range of operational states and accident conditions, including different levels of availability of the safety systems.
Uncertainties presented by alternative and innovative features can and do affect the outcomes of safety analyses.
New features may introduce additional phenomena and behaviours that systems may need to address. This means that such features must be supported through experimental results and use of validated computer codes. This is to properly model them, as well as the associated phenomena/behaviours, in the overall safety analysis of the facility at a specific location. The use of multiple levels of these features compounds the effects and may impact how and when, for example, probabilistic safety assessment is used.
One of the areas currently being examined by CNSC is the implications of PSA multiple-unit sites as well as how human actions may influence specific aspects of PSAs. The CNSC is also aware that proponents may propose alternative methodologies for safety analysis. The Canadian approach allows for this type of approach, but proponents are encouraged to engage with the CNSC as early as possible to discuss the acceptability of alternative approaches.
B.9 Additional information on defence in depth and mitigation of accidents
Defence in depth is applied to all facility states from normal operation to those beyond the design basis of the facility and through all key facility lifecycle stage activities including operation and maintenance outages through decommissioning activities. Figure 5 identifies each of the levels and explains how they are generally implemented in a project. The information presented is consistent with International Nuclear Safety Advisory Group INSAG-10, Defence in Depth in Nuclear Safety.
Level | Implementation |
---|---|
1. To prevent deviations from normal operation, and to prevent failures of structures, systems and components (SSCs) important to safety |
|
2. To detect and intercept deviations from normal operation, to prevent AOOs from escalating to accident conditions and to return the plant to a state of normal operation |
|
3. To minimize the consequences of accidents, and prevent escalation to beyond-design-basis accidents |
|
4. To ensure that radioactive releases caused by severe accidents OR design extension conditions are kept as low as practicable |
|
5. To mitigate the radiological consequences of potential releases of radioactive materials that may result from accident conditions |
|
Figure 6 depicts how those levels are integrated into the overall safety approach for a facility and this is reflected in a licensee's management systems, which include oversight over design, construction, operation, and interfaces with key external stakeholders who are part of offsite response plans.
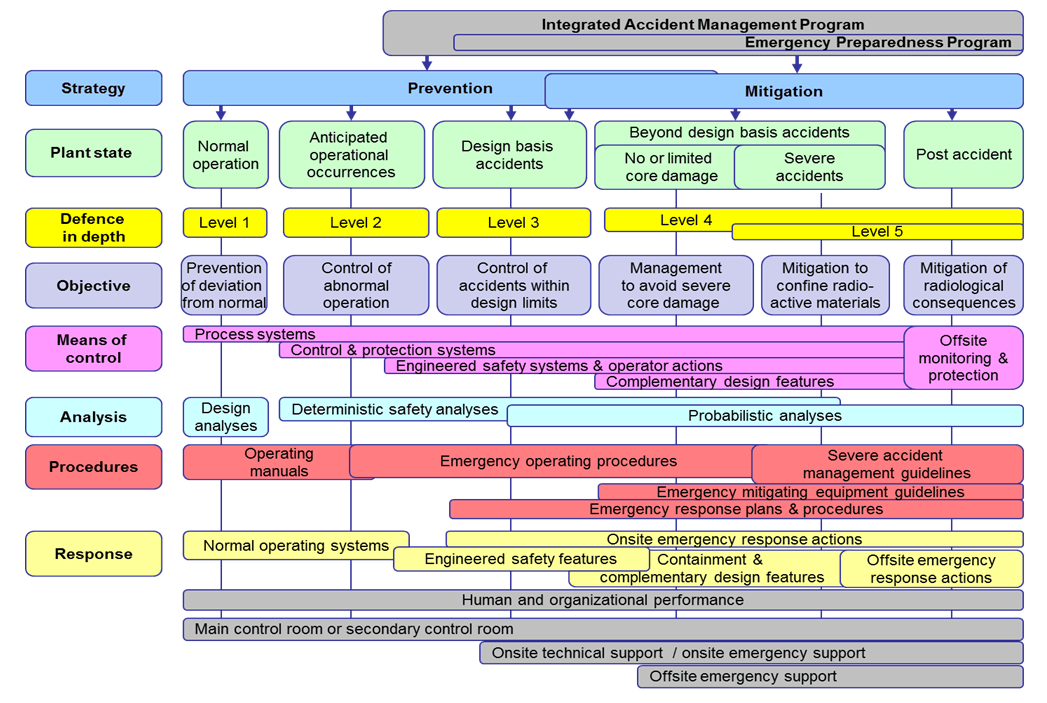
Long description
This diagram consists of a grid that is roughly aligned by plant state. For each Plant State, the Objective, Strategy, Level of Defence in Depth, Objective, Means of Control, Analysis, Procedures and Response options are listed.
For the plant state of "Normal Operation":
- the Objective is "Prevention of deviation from normal"
- the Strategy is "prevention"
- the Defence in depth is "Level 1"
- the Means of control are "Process systems"
- the Analysis is "Design analyses"
- the Procedures are "Operation manuals"
- the Response includes "Normal operating systems", "Human and organizational performance", and "Main Control room or secondary control room"
For the plant state of "Anticipated operational occurrences":
- the Objective is "Control of abnormal operation"
- the Strategy is "Prevention"
- the Defence in depth is "Level 2"
- the Means of control include "Process systems", and "Control & protection systems"
- the Analysis is "Deterministic safety analyses"
- the Procedures include "Operation manuals" and "Emergency operating procedures"
-
the Response includes "Normal operating systems", "Human and organizational
performance", and "Main Control room or secondary control room"
may utilize an "Integrated Accident Management Program"
For the plant state of "Design basis accidents":
- the Objective is "Control of accidents within design limits"
- the Strategy is "Prevention" and "Mitigation"
- the Defence in depth is "Level 3"
- the Means of control include "Process systems", "Control & protection systems", and "Engineered safety systems & operator actions"
- the Analysis includes "Deterministic safety analyses" and "Probabilistic analyses"
- the Procedures include "Emergency operating procedures", and "Emergency response plans & procedures"
- the Response includes "Human and organizational performance", "Main Control room or secondary control room", "Onsite emergency response actions", "onsite technical support / onsite emergency support", and "Engineered safety features"
- utilizes the "Integrated Accident Management Program" and the "Emergency Preparedness Program"
For the plant state of "Beyond design basis accidents - no or limited core damage":
- the Objective is "Control of core damage to avoid severe accident"
- the Strategy is "Prevention" and "Mitigation"
- the Defence in depth is "Level 4"
- the Means of control include "Process systems", "Control & protection systems", "Engineered safety systems & operator actions", and "Complementary design features"
- the Analysis includes "Deterministic safety analyses" and "Probabilistic analyses"
- the Procedures include "Emergency operating procedures", and "Emergency response plans & procedures"
- the Response includes "Human and organizational performance", "Main Control room or secondary control room", "Onsite emergency response actions", "onsite technical support / onsite emergency support", "Offsite emergency support", "Engineered safety features", and "Containment & design features"
- utilizes the "Integrated Accident Management Program" and the "Emergency Preparedness Program"
For the plant state of "Beyond design basis accidents - severe accidents":
- the Objective is "Mitigation to confine radioactive materials"
- the Strategy is "Mitigation"
- the Defence in depth is "Level 4" or "Level 5"
- the Means of control includes "Process systems", "Control & protection systems", "Engineered safety systems & operator actions", and "Complementary design features"
- the Analysis includes "Deterministic safety analyses" and "Probabilistic analyses"
- the Procedures are "Severe accident management guidelines",
- the Response includes "Human and organizational performance", "Main Control room or secondary control room", "Onsite emergency response actions", "onsite technical support / onsite emergency support", "Containment & design features", "Offsite emergency support", and "Offsite emergency response actions"
- utilizes the "Integrated Accident Management Program" and the "Emergency Preparedness Program"
For the plant state of "Post accident":
- the Objective is "Mitigation of radiological consequences"
- the Strategy is "Mitigation"
- the Defence in depth is "Level 4" or "Level 5"
- the Means of control is "Offsite monitoring & protection"
- the Analysis includes "Probabilistic analyses"
- the Procedures are "Severe accident management guidelines"
- the Response includes "Human and organizational performance", "Main Control room or secondary control room", "Onsite emergency response actions", "Onsite technical support / onsite emergency support", "Offsite emergency support", and "Offsite emergency response actions"
- utilizes the "Integrated Accident Management Program" and the "Emergency Preparedness Program"
Each reactor technology's characteristics along with where it will be located (i.e., a site) influences how the objective of each level of defence in depth is addressed. The overall safety approach used by a proponent must address both of these factors both on the site and with stakeholders in the surrounding regions.
The CNSC is aware that designers of new reactor technologies, including SMRs, are placing a greater emphasis on implementing engineered preventative measures to reduce the need to rely on mitigation measures. The general reason for doing this, in theory, is that stronger preventative measures should increase certainty around:
- reducing the probabilities of entering into accident situations that would result in significant consequences
- ensuring that such an accident would have the smallest possible consequences if it progressed
Some examples of preventative measures being proposed by SMR developers include:
- smaller reactor core inventories on a per reactor basis – to improve ability to control, cool and contain fuel during and following facility events
- new fuels with much higher robustness to withstand plant events without degrading – this would act to reduce releases
- alternative heat removal technologies to passively cool the fuel during and following an event
- alternative reactor component configurations to reduce or even eliminate high energy events associated with loss-of coolant accidents
- using greater amounts of automation to assist operational and maintenance staff with their oversight of the facility
Many of these technical measures were studied decades ago, but the technology was not mature enough to permit its use in practice at the time. Newer engineering materials and improved computational tools are leading to these measures being proposed for use again. In many cases, technical claims are being made that these designs will either reduce potential beyond-design-basis accidents (with significant consequences) to a very low probability or eliminate them altogether.
A reactor facility design is required to meet the safety objectives of all five levels of defence in depth, including physical barriers to prevent uncontrolled releases of radioactive materials to the environment. The levels of defence of depth are expected to be independent to the extent practicable. In the licensing process, a proponent will be required to demonstrate how the levels of defence in depth are sufficient in their implementation. A demonstration – such as that from research and development activities, including results from physical experiments – will be expected to include credible evidence and information. The requirements for both design and safety analysis include consideration of areas including:
- external hazards that can breach multiple levels of defence in depth simultaneously
- common-cause/mode failures that cut across the concept of independence of levels
- provenness of design tools
B.10 Background on emergency planning zones
In Canada, as depicted in figure 7, there are two primary types of planning zones:
- Exclusion zone: Per section 1 of the Class I Nuclear Facilities Regulations, an "exclusion zone" is a parcel of land within or surrounding a nuclear facility on which no permanent dwellings are allowed and over which a licensee has the legal authority to exercise control. Details on the exclusion zone are found in RD-346, Site Evaluation for New Nuclear Power Plants.
- Emergency planning zone: An emergency planning zone (EPZ) is defined as the area in which implementation of operational and protective actions might be required during a nuclear emergency, to protect public health, safety, and the environment. An EPZ addresses emergency measures to be used outside the licensee's exclusion zone and that are normally controlled and executed by an external emergency planning authority.
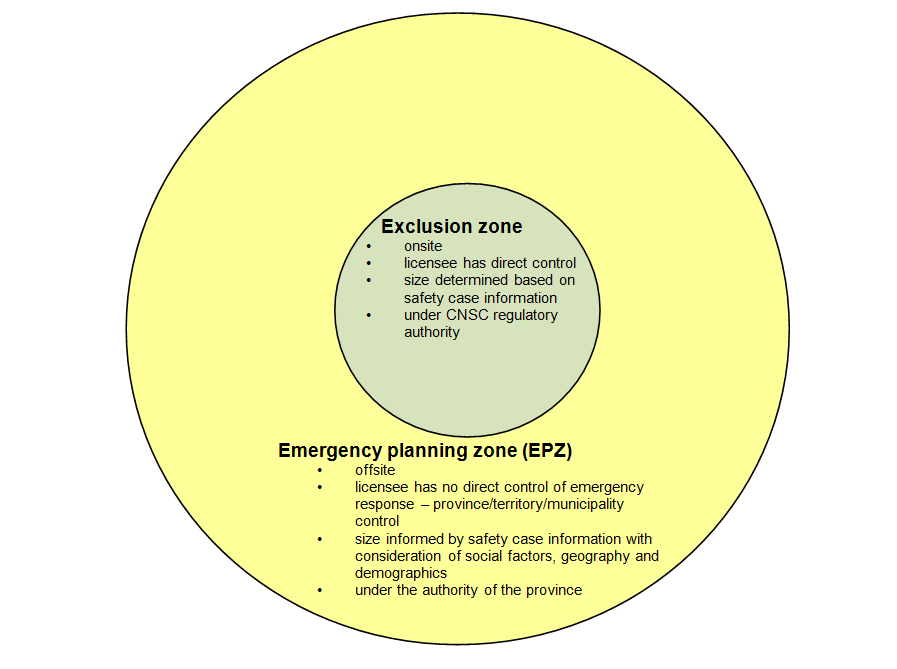
Long description
The diagram depicts the relationship between an emergency planning zone (EPZ) and an exclusion zone.
The exclusion zone is represented as a small circle in the centre of the diagram. and it normally represents the nuclear power plant site.
The emergency planning zone is depicted as a larger circle surrounding the exclusion zone.
An EPZ can be further broken down into additional subzones to address the following objectives (extracted from CSA N1600, General Requirements for Nuclear Emergency Management Programs):
- Provisions for automatic actions: A designated area (automatic actions zone [AAZ]) immediately surrounding a nuclear power plant (NPP) where planned protective actions are implemented by default on the basis of NPP conditions, with the aim of preventing or reducing the occurrence of severe deterministic effects. This includes licensee actions within the exclusion zone.
- Detailed planning: A designated area (detailed planning zone [DPZ]) surrounding an NPP, incorporating the AAZ, where planned protective actions are implemented as needed on the basis of NPP conditions, dose modelling, and environmental monitoring, with the aim of preventing or reducing the occurrence of stochastic effects.
-
Contingency planning: A designated area (contingency planning zone [CPZ]) surrounding an NPP, beyond the DPZ,
where plans or arrangements are made in advance, so that during a nuclear emergency:
- protective actions can be extended as required to reduce potential for exposure
- dose rate monitoring of deposition is conducted to locate hotspots that could require protective actions following a release
- Ingestion control planning: A designated area surrounding an NPP where plans or arrangements are made to:
- protect the food chain
- protect drinking water supplies
- restrict consumption and distribution of potentially contaminated produce, wild-grown products (can include mushrooms and game), milk from grazing animals, rainwater, animal feed
- restrict distribution of non-food commodities until further assessments are performed
These subzones are not discussed further in this discussion paper as they are considered to be part of the overall EPZ for the purposes of discussion.
B.10.1 Background on roles and responsibilities of responsible participants and agencies
Provinces/Territories
Provincial and territorial governments have primary responsibility for offsite emergency planning and response to protect public health, property and the environment. Each province prepares its provincial nuclear emergency response plan (PNERP) in coordination with the federal government, under the Federal Nuclear Emergency Plan (FNEP). For example, in Ontario, where most nuclear power stations in Canada operate, the PNERP can be found at the following link: Emergency Management Ontario: Emergency Response Plans.
Health Canada
Health Canada, as the lead department under the FNEP (Health Canada, 2002), provides guidelines for intervention following a nuclear emergency in Canada or affecting Canadians in Canadian Guidelines for Intervention During a Nuclear Emergency - November 2003.
These guidelines are a key reference for provincial governments when preparing provincial nuclear emergency plans, as well as other responsible agencies and applicants for licences for activities regulated under the Nuclear Safety and Control Act.
Canadian Nuclear Safety Commission
The CNSC is the regulatory authority for licensing, compliance and enforcement for nuclear reactor facilities in Canada. As part of the licensing process, the CNSC considers the design-basis accident dose limits and confirms that the determined exclusion zone distance is appropriate to meet all safety requirements. The CNSC works closely with the province to provide information about the nuclear facility's safety case and licensing process to help the province determine the EPZ.
Applicants for activities involving new reactor facilities
Applicants and licensees for activities involving the use of reactor facilities are responsible for submitting complete applications outlining how the site evaluation and chosen technology will, through their safety analysis, result in appropriate exclusion zone and emergency response plans to meet provincial requirements.
B.10.2 Background on considerations for determining emergency planning zones
Physical design of reactor facility
CNSC licence application guides identify information that should be submitted to support a licence application, and address the submissions and level of detail needed to address the above regulations for each licensing phase. Other supporting regulatory documents for this area can be found on the CNSC website and would be applied to demonstration reactor facilities in Canada as necessary. Several regulatory documents also contain requirements and guidance that influence the information submitted by an applicant to support exclusion zone and EPZ decision making. Other regulatory documents speak to information needed to support the facility safety case, including evidence to support provisions in level 5 of defence in depth as well as confidence in the ongoing safe operation of the facility.
Reactor vendors consider the range of applications and environments as well as the regulatory requirements, for all countries of commercial interest, when developing their designs. The entire process of ensuring an appropriate exclusion zone starts with the design of the reactor facility and the design data that supports safety claims. Vendors must ensure that their designs are robust enough to meet intended safety objectives, protective action limits and to address all these potential conditions.
Postulated initiating events
Postulated initiating events (PIEs) are theoretical events that can cause one or more adverse effects on the facility. They form a key input to the safety analysis of a facility design in all of its potential environments. These events consider internal events, such as the breaking of an installed component within the plant, or an electrical fire as well as external events such as a significant earthquake or flooding. Vendors, owners groups, regulators, researchers and other nuclear safety organizations are involved in developing and maintaining the body of practice around determination of PIEs.
Information about PIEs inform deterministic and probabilistic safety assessments (see section 3.9)
Limiting credible accident and criteria for identification of the planning accident
Based on the safety analysis, applicants/licensees would identify a list of limiting credible accidents. It is their responsibility to propose the planning basis, taking the guidance into account when selecting the limiting credible accidents. Requirements for planning basis are articulated in REGDOC-2.10.1, Nuclear Emergency Preparedness and Response. The applicants/licensees are required to provide the necessary information (credible limiting accidents and associated source terms) for the provincial and regional authorities to effectively establish their emergency planning policies and procedures. This includes the establishment of the provincial planning accident and the eventual establishment of the EPZ.
Source term and releases
The resulting source term is a list of all of radionuclides that would be released to the environment for the set of accidents, following the functioning of all the safety systems to their expected performance under accident conditions. The source term also includes the release duration and other parameters, such as the height at which a release may occur.
Considerations of meteorology for atmospheric dispersion and deposition models
The site's meteorological characterization data and modelling is applied to the release to see how the types of isotopes would travel through the air and be deposited throughout the environment.
Dose assessment and distribution in consideration of pre-established dose criteria
Once the dispersion and deposition models have been characterized, the resulting exposure pathways and dose calculations are performed. These are assessed against pre-established dose criteria for emergency response measures to determine the distances to which certain protective actions such as sheltering and evacuation would be required.
Assessment of other external factors
Other external factors are then applied. These factors have requirements for their proper consideration in the planning basis or for security reasons that may require adjustments to the EPZ. These considerations take into account for example, security, town limits, the emergency response plans, social factors as considered through the public EA and licensing process.
EPZ determination process map
Figure 8 illustrates the overall process for determining EPZ extent in Canada. It is important to note that several responsible federal and provincial/territorial agencies are involved, as indicated earlier in this section, according to their mandates and respective roles and responsibilities.
It is important to note that the applicants/licensees are required to provide regional and provincial offsite authorities with the necessary information to allow for effective emergency planning policies on a periodic basis. This requires the applicants/licensees to provide information to the provincial authorities that would help the province establish the appropriate EPZ around the nuclear facility. This information may include the limiting credible accidents and their associated source terms. From the list of limiting credible accidents, the province will determine the planning accident based on established criteria.
The resulting source term from the selected accidents would be used with the sites meteorological characterization data and modelling to determine the isotopic dispersion. Once the dispersion and deposition models have been characterized, the resulting exposure pathways and dose calculations are performed. These are assessed against pre-established protective action limits (PAL). PALs are dose criteria for emergency response measures to determine the distances over which certain protective actions such as sheltering and evacuation would be required. In addition, the provincial authorities would also consider social factors, geography and demographics in determining the EPZ around the nuclear facility. Although the determination of the EPZ size is under the province's authority, the province works with multiple supporting organizations to develop a technical planning basis that would be used to determine the EPZ. In summary, the EPZ extent is based on the nuclear reactor's technology, the resulting dose assessments against the provincial PALs, and various external factors such as social considerations, demographics and geography. Figure 9 depicts the process for determining the extent of an EPZ.
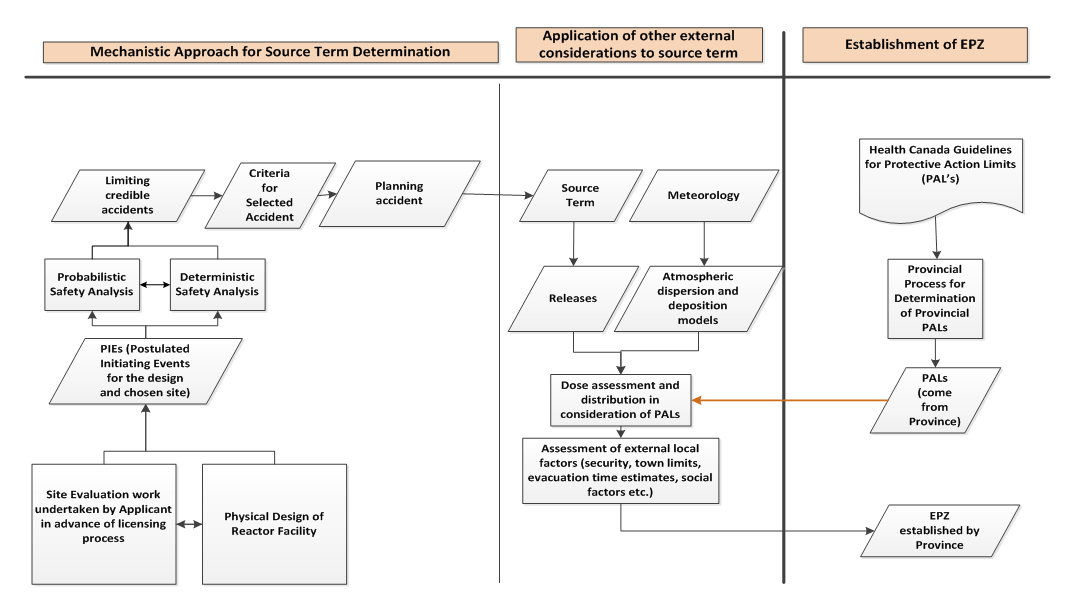
Long description
The diagram depicts a flow diagram for determining EPZ extent in Canada, as described in section B10.2.
B.10.3 Background on transportable reactor concepts
Vendors have noted that for smaller facilities located in remote regions, onsite reactor refuelling may be inefficient from a logistics perspectiveFootnote [25] and may be undesirable from a public acceptance perspective. As a result, vendors are developing concepts that:
- extend the operating time of a reactor between refuelling – this can be accomplished using a combination of slightly higher fuel enrichments (while remaining within the less than 20 percent limit for low enriched uranium fuel) and operating the reactor at lower power levels; this would mean that a reactor would be able to operate without refuelling for between 5 and 30 years dependant on the designFootnote [26]
- do not require onsite refuelling – these designs would be fuelled and sealed at the factory of origin and delivered to the site for installation; spent sealed modules would be removed from the site and sent to a central location (i.e., factory of origin) for refurbishment/refuelling or interim storage pending decommissioning
Figure 9 illustrates a possible process that might be proposed by a vendor for deployment of such transportable SMR designs intended for deployment on land-based sites. In other parts of the world there are efforts being made to develop both surface marine (Russian Federation, China, USA) and sub-surface marine (France, Russian Federation) power plants. In both cases, these vessels would not be nuclear propelled as is the case for nuclear icebreakers or submarines, but rather marine platforms for nuclear power plants. To date, no marine designs have been proposed for possible use in Canada, so the CNSC has been focusing on small land-based designs.
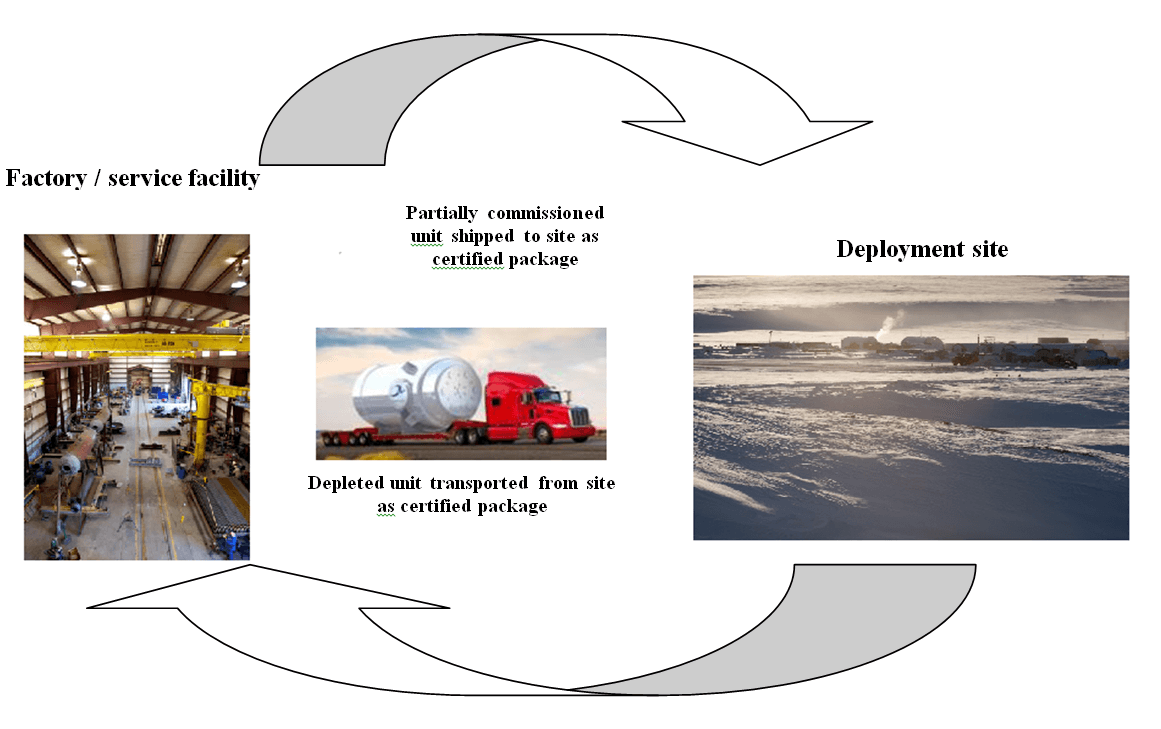
Long description
Figure 9 illustrates the transport of a reactor from a modern factory to a small village in northern Canada.
Primary elements in the above process
Deployment site: This is where the facility would be ultimately assembled and placed into service. Such a facility would be built and operated under the traditional licensing process for nuclear power plants (e.g., under the Class 1 Facilities Regulations). The site would likely have civil structures and supporting systems constructed in advanceFootnote [27] to support safe operation of the facility. The licensee then would procure reactor modules from the factory / service facility for installation at the site, and then oversee/perform commissioning activities before assuming operation of the facility. Once the fuel in a reactor module is considered to be spent, a new reactor module would be delivered and placed into service. The spent reactor module may remain on the site for a period of time to cool before being shipped back to the factory / service facility in a certified transport package.
Factory / service facility: Reactor module sub-components would be received here from suppliers and assembled, in a controlled environment, into a reactor module. Fresh fuel would then be loaded into the core, with limited commissioning and system integration testing done to confirm the module meets specifications and is ready for shipment. It is not known at this time whether any factory testing would result in irradiation of the fuel; however, if this is the case, additional transport requirements would apply to address the irradiated fuel, including prevention of criticality. The nature of the activities in such a facility give it the characteristics of a Class 1A Facility and therefore would follow the licensing process for nuclear plants with one key difference: the operating phase of the factory would never involve full-power operation of any reactors. From a safety perspective however the safety measures that would need to be in place would be very similar to what would be found for a small nuclear power plant. Requirements and guidance for nuclear power plants would therefore apply commensurate with risks presented by activities at the facility.
Transport phase: This phase of the deployment model represents a novelty in a number of areas because it involves:
- physical movement of a pre-configured and fuelled reactor core across a long distance – operational experience is currently limited to marine-based reactors used in ice-breakers and military vessels; the impacts of transport will need to be well understood to mitigate against damage to reactor systems that could impact on safety; the site licensee will be responsible for both assessing the as-received state of the module, in fulfilling its regulatory obligations as the consignee under the Packaging and Transport of Nuclear Substances Regulations, and its acceptability for installation and operation
- the transport of a core's inventory of irradiated fuel – there is significant operational experience in transport of spent fuel packages around the world and international safety requirements already address this; however, transport of a large quantity of irradiated fuel in a single package has not been performed in Canada and must meet the requirements of the Packaging and Transport of Nuclear Substances Regulations. It can be done safely but public acceptance is likely to be challenging.
Packaging and transport of nuclear substances in Canada:
- The CNSC web site provides comprehensive information about processes and regulatory requirements used for packaging and transport of nuclear substances, as prescribed under the Packaging and Transport of Nuclear Substances Regulations.
- As a part of an international effort being conducted by the IAEA, the CNSC is studying, from a regulatory perspective, the ongoing legal and institutional issues that need to be understood and resolved for this type of reactor deployment scenario. The IAEA Nuclear Energy Series, NG-T-3.5, Legal and Institutional Issues of Transportable Nuclear Power Plants: A Preliminary Study describes some of the challenges. The current work is establishing hypothetical case studies to understand how the step-by-step deployment would proceed and the regulatory aspects associated with deployment.
B.11 Additional information on increased use of automation for plant operation and maintenance
The trend in complex industries is to increasingly automate as many operational and maintenance functions as possible to:
- improve precision and efficiencies of processes
- improve processes such as monitoring equipment reliability 'live' rather than through periodic inspection
- reduce human errors in interfaces with the plant and improve supervisory functions by providing better-quality information
These approaches have been successfully proven in many risk-sensitive industries, including transportation, mining machinery, aerospace, rail and chemical manufacturing.
This trend is also true for nuclear power technologies of all sizes, where such approaches can result in an improved operational understanding of the plant and reduce radiological exposures to workers. SMR technologies appear to be pursuing automation more aggressively in an effort to both supplement defence-in-depth provisions while reducing staffing costs.
At the extreme end of the SMR spectrum, there are few proponents of very small designs who are investigating the long-term feasibility of fully autonomous plant operation with remote monitoring and intervention. (i.e., minimal to no onsite staff and a regional control room infrastructure to control a fleet of small plants) This approach would involve specific control and communication platform architectures that would allow the plant to perform fully automatic decision making under an automated supervisory program. The architecture would send key information to an offsite human interface system (offsite control centre) where an operator could monitor, and if necessary intervene remotely.
The approach would require a significant body of evidence to demonstrate that safety features can permit this form of operation. It would likely be limited to reactor designs with a several inherent safety features such as:
- a highly stable core (and nuclear flux) configuration
- fuel that is highly tolerant to plant events
- releases have been practically eliminated from the design through additional provisions not requiring human intervention over a sufficiently long period of time.
- the ability of the control system to predictably react on loss of communication from the offsite control centre.
This type of approach has been successfully implemented in numerous hydro-electric dams in places such as Northern Ontario. In Canada, SLOWPOKEFootnote [28] research reactors are allowed to operate unattended for a maximum period of 24 hours; however, the Operator should be able to reach the reactor within 2 hours to respond to an alarm. Corporate security is required to be onsite 24/7, and to contact the Operator in case of an alarm and follow the emergency procedure until the operator arrives. In this case, there is no use of offsite control facilities.
Safety and security objectives must be addressed
Modern technologies are likely to enable the use of extensive automation, but this strategy needs to be considered carefully in the overall safety and security objectivesFootnote [29] of the facility.
The CNSC has noted that, around the world, instrumentation and control challenges have arisen in both regulatory reviews of new technologies and licensing of projects referencing new reactors. The CNSC requires operator action to be planned with due regard for the time available for response, the physical environment to be expected, and the associated psychological demands made on the operator.
B.12 Background on waste management and decommissioning
The Government of Canada's Radioactive Waste Policy Framework (1996) is a structure of policies, legislation and responsible organizations set in place to govern the management of radioactive waste in Canada.
The federal government, including the CNSC:
- ensures that radioactive waste disposal is carried out in a safe, environmentally sound, comprehensive, cost-effective and integrated manner
- develops policy, to regulate and to oversee producers and owners, to ensure they comply with legal requirements and meet their funding and operational responsibilities, in accordance with approved waste disposal plans
In accordance with the "polluter pays" principle, waste producers and owners are responsible for the funding, organization, management and operation of disposal and other facilities required for their wastes.
The CNSC coordinates and implements policies, strategies and plans with its federal and international partners to ensure that waste owners and those possessing radioactive waste treat, handle, manage and store it safely and securely.
Commensurate with the associated radiological, chemical and biological hazards, waste management programs are required at all CNSC-licensed facilities leading up to, during and post operation. Licensees are responsible for waste minimization at the source, segregation, characterization, packaging, processing, storage, and proper disposal. It is the responsibility of waste's owner to fund, organize and carry out the necessary waste management activities. These waste management activities must reflect the fundamental safety concerns related to criticality, exposure, containment and, where necessary, retrievability.
To do this, licensees must develop a waste management program that helps to reduce the overall volume of radioactive waste requiring long-term management.
They are also expected to investigate and implement new radioactive waste management technologies and techniques as they become available. Some of these strategies include:
- reusing and recycling materials by separating radioactive components from non-radioactive ones
- preventing contamination by restricting the amount of materials in radioactive areas
- assessing technology advances in waste minimization, and implementing improvements to waste-handling facilities that reduce the volume of radioactive waste
Long-term waste management considerations for proposed projects involving SMRs
SMR proponents are responsible for planning early to ensure that suitable facilities will be available to accept and effectively manage their anticipated waste streams whether onsite or offsite. For offsite facilities concerned with spent fuel, proponents are expected to approach other agencies involved in long term waste management and disposal planning, such as the Nuclear Waste Management Organization, which has the responsibility for long-term management of Canada's used nuclear fuel. The selected approach for the long-term management of used fuel is adaptive phased management.
High-level radioactive waste at existing sites in Canada is normally stored onsite for several years in spent fuel pools before being moved to onsite dry storage facilities. For larger SMRs, the CNSC understands that this is likely to remain the case for more traditional water-cooled SMRs.
Of particular regulatory interest, however, is the concept of a factory-fueled and sealed transportable reactor module (for very small SMR designs). In theory, such a design might be a single-use (i.e., non-refuelable) reactor core / fuel cartridge designed to operate from 5 to 30 years on its load of fuel.
Shared waste management facilities for SMRs
With the possibility of SMR sites being significantly smaller, SMR licensees may seek to share facilities for interim storage and eventual disposal of both conventional hazardous and irradiated wastes including:
Such licensed facilities already exist in Ontario (see figure 10) but exist under a single licensee who establishes agreements with other sites who seek to use the facilities. In other parts of Canada, these facilities would need to be established and licensed under a single licensee.
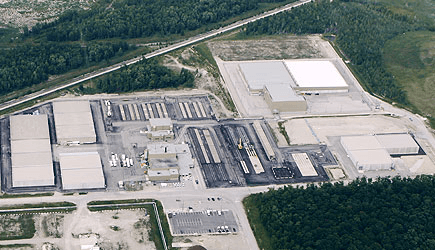
Photo courtesy of the Nuclear Waste Management Organization.
Within the CNSC's regulatory framework, it is the licensee's responsibility to determine the approach and rate of decommissioning for a facility that no longer operates. Selection and justification of the decommissioning strategy (prompt removal, deferred removal, in-situ confinement, or some combination of the above) is the responsibility of the operator, as is the timing of the activities. Recognizing that uncertainty related to new technology may drive many of these considerations and the associated regulatory reviews, the operator would be expected to plan based on the conservative information and predictions, and prepare specific work packages to describe the activities to be carried out and the associated timelines. Additionally, in selecting the final state of the site, consideration should be given to the facility's location. For example, if the facility is located centrally within a community, it may be preferable to return the site to a state suitable for community use promptly, rather than long-term deferred removal and dismantling.
B.13 Background on sub-surface civil structures important to safety
One of the key defining features of many proposed SMR designs is that the nuclear island would be located either partially or fully underground. As a concept, this idea is not new and was first envisioned in the 1950s in most of the countries that first developed nuclear technologies. In Canada, this concept was adopted for the National Power Demonstration (NPD) reactor in Rolphton and the WR-1 reactor at Whiteshell. Among the primary reasons being touted by vendors for placing a reactor building below ground are:
- added structural strength afforded by the surrounding rock – the possibility to further reduce potential for fission product release in the event of a serious reactor accident
- the facility would have a lower threat profile – e.g., aircraft crash
- easier to secure the facility from unauthorized entry (less entrances)
- improved non-proliferation – e.g. , more difficult to remove materials from the facility
- protection against the effects of most severe weather events and some potentially protection even from the effects of earthquakesFootnote [30]
- use of natural radiation shielding materials (rock and soil)
One of the primary reasons why this approach was not adopted early in the days of nuclear technology development was the high cost of excavation and stabilization of subterranean chambers versus the lower costs of above-ground concrete and steel structures. However, technological advances in drilling, tunneling and excavating large subterranean spaces are leading designers to re-examine the use of subterranean engineered structures in their safety cases.
Figure 11 depicts a cross-section of a hypothetical SMR design with a reactor building located partially below grade, such that the reactor module is below ground but the roof of the common confinement / maintenance building is above grade to permit access for maintenance activities. Very small SMR concepts for remote regions are also considering the use of subterranean pre-engineered silos/vaults that would be factory fabricated for more efficient site installation.
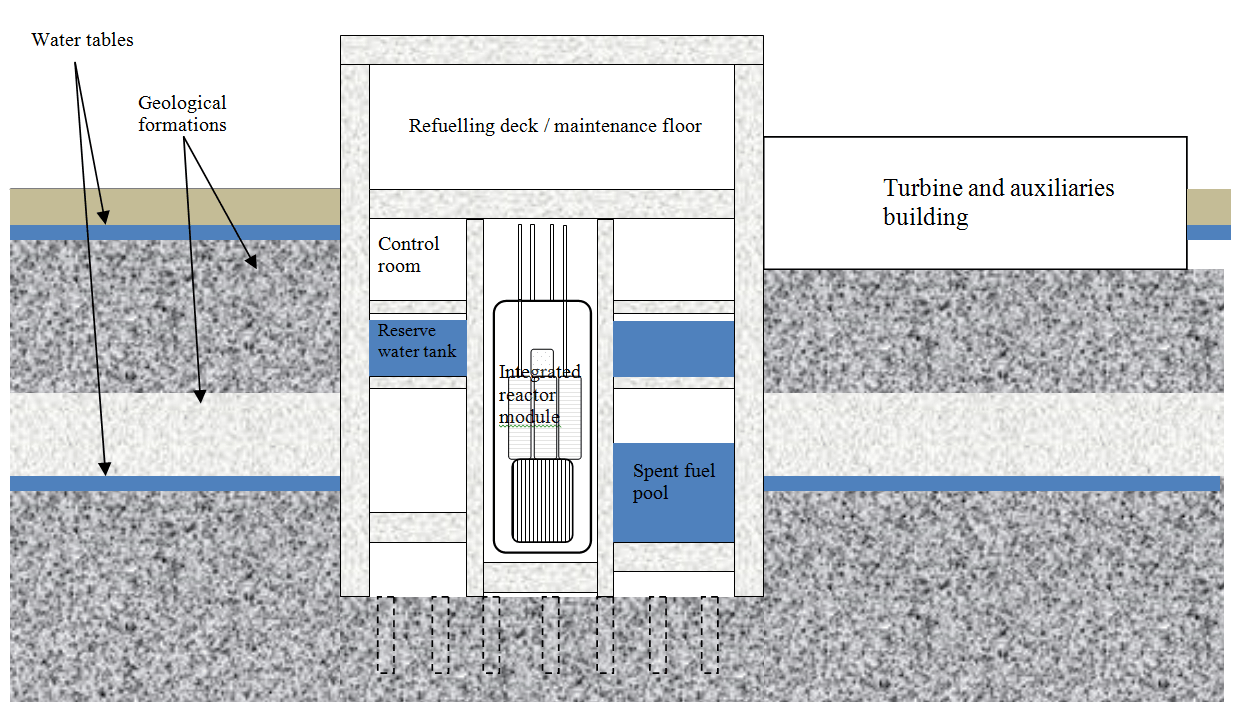
Long description
Figure 11 depicts a very simple cross section diagram of a reactor building and balance of plant for a hypothetical SMR.
Canadian and American codes and standards for nuclear structuresFootnote [31] do not currently contain provisions to address deeply embedded structures. CNSC is gathering the information to bridge the gap. For example, CNSC specialists:
- are working with US-Nuclear Regulatory Commission staff and the Organization for Economic Cooperation and Development (OECD)- Nuclear Energy Agency research program to gather information in this area to inform future work on standards
- have a research program in place with the University of California at Davis to address acceptance criteria for seismic soil-structure interaction for embedded structures
- have a research program in place with Purdue University to address gaps in standards for steel plate to concrete structures
Appendix C: Consolidation of questions
The following questions are asked within this document and repeated below for convenience.
From section 2.2:
For the topic of "technical information, including research and development activities used to support a safety case", are requirements regarding the scope and adequacy of supporting information sufficiently clear?
Of particular interest are whether existing R&D requirements are clear in key regulatory documents such as REGDOC-2.5.2, Design of Reactor Facilities: Nuclear Power Plants, RD-367, Design of Small Reactor Facilities, REGDOC-2.4.1, Deterministic Safety Analysis, and other documents related to facility lifecycle (e.g., REGDOC-2.6.3, Fitness for Service: Aging Management).
From section 2.3:
For the topic of "Licensing process for multiple module facilities on a single site", are clarifications needed to REGDOC-3.5.1, Licensing Process for Class I Nuclear Facilities and Uranium Mines and Mills?
In order to be better prepared for the use of replaceable reactor core modules or relocatable facilities, the CNSC is seeking information on facility deployment strategies being considered by developers, including impacts of such an approach on areas such as worker and public safety, environmental assessment and decommissioning.
The CNSC will use this information for future more-detailed workshops to discuss regulatory implication of different deployment approaches.
From section 2.4:
For the topic of "Licensing approach for a new demonstration reactor", is there a need for additional clarification or information beyond that found in RD/GD-369, Licence Application Guide: Licence to Construct a Nuclear Power Plant? If yes, what needs to be clarified or added?
With respect to addressing uncertainties introduced by the application of integrated multiple novel features in a demonstration facility, are requirements regarding the scope and adequacy of supporting information sufficiently clear?
What, if any, requirements need to be revisited to address activities involving demonstration reactors? For example, are additional requirements or guidance needed to address operational restrictions if the facility is being used to gather operating experience that would be normally be needed for commercial facility licences?
From section 2.5:
For the topic of "licensing process and environmental assessments for fleets of SMRs", how do you envision proposals for such fleets across large geographical territories proceeding through licensing and environmental assessments?
How would the principles discussed in REGDOC-3.5.1, Licensing Process for Class I Nuclear Facilities and Uranium Mines and Mills be applied and where might challenges exist?
From section 2.6:
For the topic of "Management system considerations: Licensees of activities involving SMRs", to help the CNSC prepare for alternative ownership and operating models that would be used in SMR deployment, more details (such as case studies) are sought regarding areas including:
- how deployment of different SMR concepts (e.g., factory fueled transportable concepts) would proceed
- how oversight for such deployments would be conducted
- how issues such as licensee performs inspections of key components (e.g., a reactor module) when received from a vendor
- how alternative ownership models will address requirements in CSA Group's standard N286‑12, Management system requirements for nuclear facilities and in CNSC regulatory requirements
The CNSC will use this information for future, more detailed workshops to discuss regulatory implication of different deployment approaches.
See section B.6 of appendix B for additional background information.
From section 2.6.1:
For the topic of "management system: minimum complement in SMR facilities", are the regulatory requirements and guidance related to minimum complement sufficient and clear as applied to activities involving SMRs? What, if any, proposed changes should be considered for the existing regulatory requirements? For example, in conjunction with the question in section 2.12, is additional guidance needed to address human coverage for failure of automated systems?
From section 2.7:
For the topic of "safeguards implementation and verification", the CNSC would like to hear if its current framework provides enough clarity to effectively ensure safeguards verification of novel fuels and new designs.
From section 2.8:
For the topic of "deterministic/probabilistic safety analyses", are the regulatory requirements and guidance clear for the kinds of alternatives that might be proposed for Deterministic/probabilistic safety analyses for SMR facilities? Do the existing requirements permit the establishment of a suitable level of probabilistic safety analysis for different novel designs?
Does enough information currently exist to apply probabilistic safety analysis to novel designs?
From section 2.9:
For the topic of "defence in depth and mitigation of accidents", given some of the novel safety approaches that vendors are proposing, are the existing requirements and guidance around defence in depth adequately clear for prevention and mitigation of accidents? Consider this question with particular attention to the following topics and combinations thereof:
- application of inherent and/or passive safety features
- application of alternative instrumentation and control strategies (e.g., remote monitoring and intervention of a fully-automated facility)
- non-water cooled technologies
- transportable sealed and factory fueled SMRs (see section 2.11)
- facilities proposed to be located in highly remote regions
From section 2.10:
For the topic of "emergency planning zones", are the requirements and guidance related to EPZs sufficiently clear to enable an organization to submit a licence application for a facility-specific EPZ while still meeting the CNSC's expectations regarding the environment and worker health and safety?
Are there specific considerations that need to be incorporated into requirements and guidance for specific siting cases like remote regions?
From section 2.11:
For the topic of "Transportable reactor concepts", the CNSC is seeking information about deployment scenarios for further discussion. Examples of questions to inform future discussions include:
- How might deployment of such concepts proceed? (The CNSC seeks examples such as case studies.)
- What nature of activities will occur at the factory or service facility versus the site and how will those activities interface with one another from a management-system perspective?
- What would environmental impact statements look like?
- What would the relationship between the manufacturing facility, the facility fuelling the reactor modules, the carrier transporting the modules and the site operator entail?
- How would post-shipment inspections be conducted and addressed by the licensee of the deployment site facility?
- How would these scenarios be impacted if major components or modules were imported or exported?
- How would transport be conducted such that transport requirements would be met throughout the deployment journey?
- What is the strategy for performing safety analysis for all deployment activities?
- How would these scenarios be impacted if major components or modules were imported or exported?
- How would transport be conducted such that transport requirements would be met throughout the deployment journey?
- What is the strategy for performing safety analysis for all deployment activities?
From section 2.12:
For the topic of "increased use of automation for plant operation and maintenance", is additional clarity needed in existing requirements and guidance related to the implementation of automation strategies for SMRs?
Specific to autonomous operation with remote monitoring and intervention, what safety and control measures could be taken to help prevent/mitigate communication loss between the SMR and the monitoring facility?
From section 2.13:
For the topic of "human/machine interfaces in facility operation", the CNSC is seeking comments from
technology developers who are proposing new HMI technologies approaches/architectures for use in SMRs.
Is additional clarity needed in existing requirements and guidance for HMIs used for facility operation and maintenance? If so, what areas could benefit from additional clarity?
From section 2.14
The CNSC is currently working on a separate discussion paper on human performance and will request feedback on this specific topic in the near future.
For the topic of "The impact of new technologies on human performance" is additional clarity needed in existing requirements and guidance for human performance in an SMR environment?
From section 2.15:
For the topic of "financial guarantees for operational continuity", is additional clarity needed in existing requirements and guidance related to the implementation of financial guarantees for operational continuity to ensure safe termination of licensed activities?
Are there other financial instruments not listed in G-206 that would be useful in helping put financial guarantees in place?
From section 2.16:
For the topic of "site security provisions", what regulatory issues may present challenges to deployment scenarios for SMR facilities? For example:
- How could subsurface or civil structures be implemented as part of the security by design approach?
- How might security provisions differ for SMRs with a very limited onsite staff and located in a remote region? How would possibly lengthy offsite response times be addressed?
- How would security provisions be addressed for offsite monitoring/control of facilities if used?
From section 2.17:
For the topic of "waste management and decommissioning", what are some of the key strategies for waste management, spent fuel management and decommissioning that the CNSC and licensees need to consider for various SMR deployment scenarios? For example, for companies considering a fleet of SMRs across a wide geographical area, how would waste and decommissioning be addressed?
In implementing these strategies, where are the challenges that exist in interpretation of current requirements and guidance?
From section 2.18:
For the topic of "subsurface civil structures important to safety", to complement the CNSC's investigation into ageing management of civil structures, where is SMR industry work is being performed in this area to address aging management issues in codes and standards? Of particular interest is ongoing work being done on technologies necessary to reliably demonstrate that such structures remain fit for service over the life of the facility including provisions for safe storage and decommissioning plans?
From section 3:
For the topic of "fusion technologies", what are the types and magnitudes of risks and hazards that would be posed by different fusion technologies (conventional and radiation hazards)?
With this in mind, how would the risks posed by activities involving fusion reactors differ from current nuclear fission reactors? Should fusion reactors be regulated differently than fission reactors?
Appendix D: Specific Drivers for Regulatory Readiness
Note: The information supplied in this section is simply for context and does not provide or imply any opinion on the part of the CNSC with regards to energy or nuclear policy.
The following information is used with the permission of the providers and includes statements from some industry and government entities regarding their interest in SMRs. As such, they are drivers for regulatory readiness by the CNSC.
SaskPower
"To ensure SaskPower's electricity system is able to keep pace with the province's growing demand for power and the need to retire aging generation assets, the corporation regularly undertakes an evaluation of the full range of electricity supply options to determine the best mix of power generation options for the Province of Saskatchewan. This includes conventional baseload technologies (such as cogeneration, natural gas, nuclear power and hydro), renewable options (such as wind, solar and biomass), as well as emerging technologies (such as carbon capture and storage, geothermal, nuclear power from small modular reactors) combined with the development of energy efficiency and conservation programs. Through this evaluation SaskPower will determine the clean energy portfolio that best enables the delivery of safe, reliable, sustainable power to the people of Saskatchewan at the lowest possible cost.
In 2009, with the anticipation of new federal regulations restricting the future use of coal-fired power generation, Saskatchewan's primary source of baseload power for over 50 years, SaskPower began a more detailed assessment of nuclear power, a technology that has been evaluated in Saskatchewan since the 1970s. Large sized reactors (with 'large' being defined as units >700MW) were ruled out at an early phase primarily due to financial and technical reasons related to the relatively small size of the provincial electricity grid (SaskPower's largest single contingency is 350MWe). In 2012, the corporation began a detailed assessment of the technical, economic and regulatory feasibility of nuclear power from small modular reactors (SMR) with 'small' being defined as a units < 300 MW.
The initial phase of SaskPower's SMR Feasibility study, which ended in 2015, concluded that SMRs are a very promising technology that could play a significant role in Saskatchewan's power future. Several advanced SMR technologies were identified globally that, unlike large-scale nuclear reactors, could be accommodated within Saskatchewan's small grid provided that (1) SaskPower not take first of a kind technology risk, and that (2) an SMR could be licensed, constructed and commissioned in Saskatchewan by 2030. Furthermore, based on initial cost estimates for the construction and operation of SMRs from leading SMR vendors around the world, SMRs could be an economically feasible supply option to supply clean, reliable, baseload power for the Province of Saskatchewan. This technology continues to be included in SaskPower's ongoing evaluation of a range of potential baseload options."
Sylvia Fedoruk Centre for Nuclear Innovation
"The Sylvia Fedoruk Canadian Centre for Nuclear Innovation (not-for-profit subsidiary of the University of Saskatchewan) was established with funding from the Government of Saskatchewan in 2011 to develop the capacity to support a vibrant nuclear industry in the province through advancing Saskatchewan's role in nuclear research, development and training. The Fedoruk Centre, under its mandate, is investigating both large grid connectable SMRs and very small SMRs for deployment in edge-of-grid and off-grid regions (for example for mines and northern communities). It is also facilitating academic studies in societal acceptance of nuclear energy and investigating potential spin-off- benefits of possible SMR industries in the province. A program to develop an understanding of the issues that affect the siting of SMRs using the Province as a model is in development. The Centre sees the opportunity for the Province to assist in the avoidance of greenhouse gas production around the world by developing a package of "soft services", including public policy, legal and siting to enable previously non-nuclear jurisdictions to move ahead with an SMR based nuclear program. The Centre has been visible at SMR presentations around Canada."
Government of Ontario
"The Ontario Ministry of Energy has retained third-party expertise to prepare a feasibility study regarding potential opportunities for small modular reactors (SMR) in Ontario. The study is being co-sponsored with the federal government's Department of Natural Resources.
The objective of the feasibility study is to assess the current state of SMRs in order to identify potential benefits and risks associated with their use. In addition, the study will assess the licensability of current designs in Canada, availability of funding to advance development of various designs and the estimated time required to achieve commercial operation. A final report is expected to be submitted by spring 2016."
Canadian Nuclear Laboratories (CNL)
"Canadian Nuclear Laboratories (CNL) is uniquely positioned to play a leading role in establishing SMR technology in Canada. CNL has more than 60 years of experience in nuclear Research & Development as well as operating research reactors and managing radioactive waste. CNL has many unique facilities and capabilities at the Chalk River site supported by over 3,000 staff. Some of the facilities include hot-cells, post-irradiation examination services, materials research, reactor physics analysis, thermalhydraulics facilities and analysis tools, accident consequence analysis, transportation of radioactive materials, instrumentation and control, design of specialized tools and components for nuclear applications, etc...
Siting a first of a kind SMR at Chalk River will save vendors years of regulatory effort and expense compared to other Canadian and international options. The site has well-developed security and emergency preparedness programs as well as many years of experience in licensing and operating research reactors."
Natural Resources Canada
"The Government of Canada sees a future where a clean environment and a strong economy go hand in hand, and I believe that Canada's nuclear industry — among the world's best — can and must be a big part of that. Canada is renowned around the world as a stable, reliable and cost-effective supplier of energy resources, technology and services, and our government will continue to promote our expertise in clean and safe nuclear power — and work with partners, including China — to ensure that these efforts translate into real opportunities for our nuclear industry."
Kim Rudd
Parliamentary Secretary to the Minister of Natural Resources
Emissions Free Energy Working Group (EFE-WG)
"This industry association is a Working Group of the Canadian Nuclear Association (CNA), representing SMR and Very Small Modular Reactor (VSMR) proponents with a focus on the smaller edge-of-grid and off-grid designs. While CNA represents Canada's nuclear industry at the level of policy and government relations, EFEWG draws on its members to provide expert knowledge on technical and regulatory issues. EFEWG is currently Chaired by Neil Alexander, Executive Director of the Saskatchewan-based Sylvia Fedoruk Centre for Nuclear Innovation.
CNA supports EFEWG's work through both co-funding and in-kind contributions. EFEWG aims to facilitate development of common industry positions on a variety of issues related to regulation and deployment of SMRs. The EFEWG has engaged with government agencies such as NRCan and the Ontario Ministry of Energy, and plans to approach CNSC with position papers on various regulatory areas for discussion. EFEWG contacts are Roger Humphries, EFEWG Executive Director (roger.humphries@amecfw.com) and John Stewart, CNA Director of Policy and Research and EFEWG Secretary (stewartj@cna.ca )."
Canadian Nuclear Partners (CNP) / Ontario Power Generation
"OPG, and its wholly owned subsidiary Canadian Nuclear Partners (CNP), have been supporting SMR development for over 4 years including as active participants in the B&W mPower SMR program, regular interactions with the CNSC and the Emission Free Energy Working Group and attendance at SMR industry conferences and events. Currently OPG has established an internal advisory working group to perform technical due diligence of SMR technologies and to establish and present OPG's views on required regulatory changes to support SMR deployment while maintaining stakeholder confidence in the regulatory framework for existing nuclear facilities. CNP is actively engaged with multiple SMR vendors to provide commercial based services to support their pre-licensing activities within Canada."
Bruce Power Limited Partnership
"As an operator, Bruce Power is always interested in the potential to deploy new reactor technologies. However before these new designs can be assessed for potential deployment opportunities, it is critical that operators understand the regulatory philosophy and implications in order to fully evaluate the business case. As such Bruce Power supports the CNSC's efforts to initiate discussion on the regulatory framework necessary for SMR operation.
Footnotes
- Footnote 1
-
Articulated in the CNSC's regulatory document P-299, Regulatory Fundamentals.
- Footnote 2
-
All power plants have capabilities to load-follow, but for NPPs, this load following has been limited by the reactor's ability to adjust power with time. SMRs are being developed to make rapid power adjustments to behave similarly to a gas turbine. This rapid response is necessary for very small grids, grids with rapid load changes, and those serviced by intermittent supply sources.
- Footnote 3
-
However, in 1974, the National Research Universal (NRU) reactor vessel was replaced.
- Footnote 4
-
In contrast, prototypes are generally differentiated from demonstration facilities in that prototypes are usually smaller, not fully functional and may or may not include nuclear systems. They are also designed only to perform complex experiments and test novel approaches but not to demonstrate commercial operation.
- Footnote 5
-
Response time by plant operators.
- Footnote 6
-
See appendix B, section B-9 for more information on levels of defence in depth
- Footnote 7
-
State-based control is a plant automation control design based on the principle that all process facilities operate in recognized, definable process states that represent a variety of normal and abnormal conditions.
- Footnote 8
-
These categories are further defined in the Nuclear Security Regulations schedule 1 by type, form and quantity.
- Footnote 9
-
This document contains prescribed information and is not available to the public.
- Footnote 10
-
This document contains prescribed information and is not available to the public.
- Footnote 11
-
The very small designs use higher enrichments in concert with very compact designs in order to maximize the ability to both transport a reactor using a standard nuclear transport vessel and to maximize fuel use and life in a sealed core.
- Footnote 12
-
This is a 20-MWe demonstration power reactor (decommissioning pending).
- Footnote 13
-
This was a 60-MWth reactor (decommissioning pending) to gather information for a future organic cooled CANDU concept.
- Footnote 14
-
For example, CSA Group standards N287.3 and N291.08, the American Society of Mechanical Engineers' standard BPVC Section III – Rules for Construction of Nuclear Facility Components – Division 2-Code for Concrete Containments, and the American Concrete Institute's Code Requirements for Nuclear Safety Related Concrete Structures (ACI 349-01).
- Footnote 15
-
Ref: Princeton University, Andlinger Centre for Energy + the Environment: Nuclear Distillate Article on Small Modular Reactors referrer
- Footnote 16
-
See INFO-0795, Licensing Basis Objective and Definition, January 2010, CNSC ISBN 978-1-100-14820-5.
- Footnote 17
-
A "unit" in Canada is understood to include a reactor and balance-of-plant pairing within a larger station.
- Footnote 18
-
Prototypes are generally differentiated from demonstration facilities in that prototypes are usually smaller and not fully functional systems to perform complex experiments and test novel approaches.
- Footnote 19
-
Examples of conservative design are: more robust containment, additional shutdown systems, use of supplemental emergency support systems and more on site operations and maintenance staff
- Footnote 20
-
No lower power limit exemptions are articulated in the CEAA 2012 for smaller facilities.
- Footnote 21
-
A facility may be composed of multiple units. EAs would be conducted on the maximum capacity expected for the site in the long-term regardless of the number of units to be built in the short term.
- Footnote 22
-
Note: The CNSC's mandate also includes conventional safety, security, safeguards and environmental risks in addition to radiation risks.
- Footnote 23
-
Technologies, such as specialized instrumentation, are being developed to perform such tasks remotely.
- Footnote 24
-
Information derived from REGDOC-2.5.2, Design of Reactor Facilities: Nuclear Power Plants. and REGDOC-2.4.1, Deterministic Safety Analysis.
- Footnote 25
-
Refueling activities requires special facilities and expertise to be available at each site to safely conduct refueling and manage the spent fuel.
- Footnote 26
-
Provided that the licensee can demonstrate safe operation of such a design in the face of aging mechanisms. The fuel must be able to withstand a longer residence time in-core.
- Footnote 27
-
E.g., civil structures to house the reactor module, turbine building/steam plant, cooling towers, electrical infrastructure, control room.
- Footnote 28
-
Safe LOW-POwer Kritical Experiment (SLOWPOKE) is a 20-kW thermal low-energy, tank-in-pool type nuclear research reactor designed by Atomic Energy of Canada Limited in the late 1960s.
- Footnote 29
-
The category of nuclear materials on the site also plays a role in determining the staffing levels including whether a facility can be unmanned from a security or automation perspective.
- Footnote 30
-
However, flood mitigation may require more attention depending on the site.
- Footnote 31
-
For example, CSA N287.3, CSA N291.08, ASME Section III Division 2 and ACI 349.
Page details
- Date modified: