Radiation Health Effects
What is safe?
The word “safe” means different things to different people. For many, the idea of being safe is the absence of risk or harm. However, the reality is that there is a level of risk in almost everything we do.
For example, speed limits on roads are set to maximize safety. Nevertheless, accidents occur even when drivers are obeying the speed limit. Despite the risks, we make a conscious decision to drive.
Similar conscious decisions are made when radiation is used. Radiation exposure carries a health risk. Knowing what the risks are helps the CNSC and other regulatory bodies set dose limits and regulations that limit exposure to an acceptable or tolerable risk (some may even say a safe limit).
One significant advantage with radiation is that more is known about the health risks associated with it than with any other chemical or otherwise toxic agent. Since the early twentieth century, radiation effects have been studied in depth in both the laboratory and among human populations. Since the establishment of the United Nations Scientific Committee on the Effects of Atomic Radiation (UNSCEAR) in 1955, the mandate of the Committee has been to undertake broad assessments of the sources of ionizing radiation and its effects on human health and the environment. Those assessments provide the scientific foundation used in formulating international standards for the protection of the general public and workers against ionizing radiation. The UNSCEAR 2010 Report (PDF, source: UNSCEAR Web site), the 2012 White Paper (PDF, source: UNSCEAR Web site), and the 2017 Report (PDF, source: UNSCEAR Web site) consolidates and summarizes, in simple terms, the Committee’s detailed understanding of the low-dose radiation effects on health.
How radiation affects cells
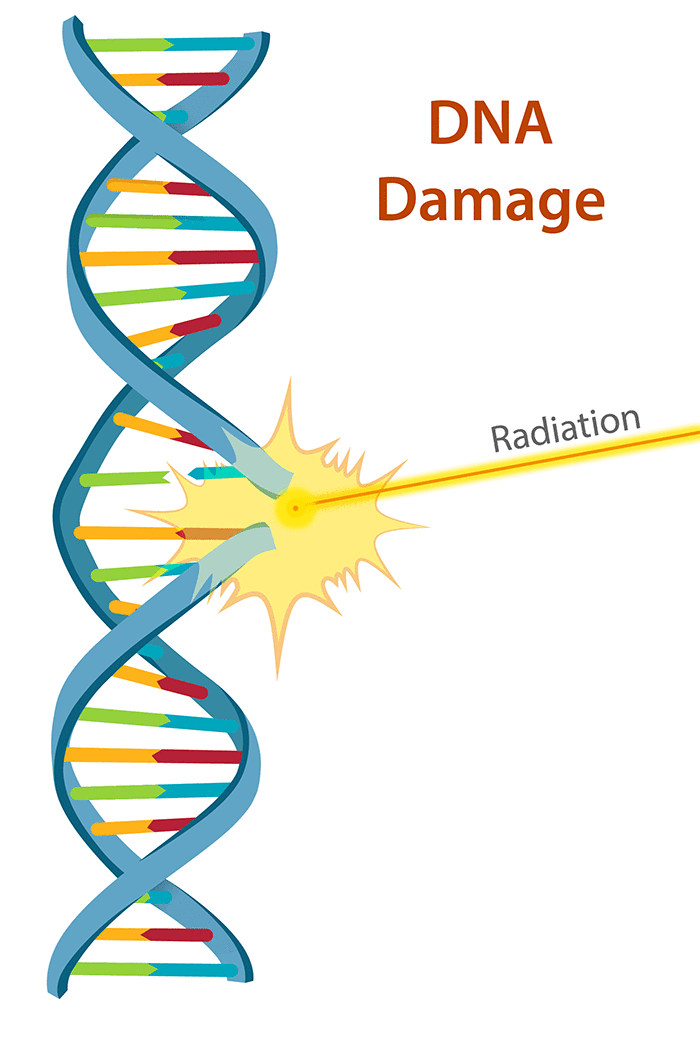
This image shows a beam of radiation breaking the double helix of a DNA strand resulting in DNA damage.
The primary way radiation affects our health is through breakage of DNA molecules. DNA consists of two long chains of nucleotides twisted together into a double helix; it is the molecular compound in the nucleus of a cell that forms the blueprint for the structure and function of the cell. Radiation is able to break these chains. When it does, three things can happen:
1) The DNA is repaired properly
In this case, the cell is repaired properly and it continues to function normally. DNA breakage occurs normally every second of the day and cells have a natural ability to repair that damage.
2) The DNA damage is so severe that the cell dies (deterministic effects)
When the DNA or other critical parts of a cell receive a very high dose of radiation, normally delivered over a short period of time, the cell may either die or be damaged beyond repair. If this kills a large enough number of cells in a tissue or organ, early radiation effects may occur. These are called deterministic effects and the severity of the effects varies according to the radiation dose received. They can include acute radiation syndrome, skin burns, loss of hair, and in extreme cases, death. Most deterministic effects occur shortly after exposure and above dose thresholds specific to each exposed tissue. The pattern of the symptoms for most of the effects is so specific that trained medical professionals can diagnose a deterministic effect of radiation.
The first evidence of deterministic effects became apparent with early experimenters and users of radiation. They suffered severe skin and hand damage due to excessive radiation dose. More recently, this relationship was observed at the 1986 Chernobyl nuclear plant accident where more than 130 workers and firefighters received high radiation doses (800 to 16,000 mSv), and suffered severe radiation sickness. Two of the people exposed died within days of exposure. Close to 30 more workers and firefighters died within the first three months.
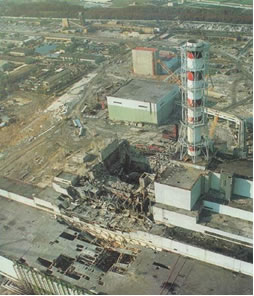
Site of the Chernobyl nuclear power
plant accident (Ukraine)
The CNSC and other international regulators put measures in place, including stringent dose limits and radioactive source tracking databases, to mitigate the chances of the public or workers receiving doses of radiation high enough to cause deterministic effects. The CNSC also has strict regulations on how nuclear substances and devices must be handled in Canada.
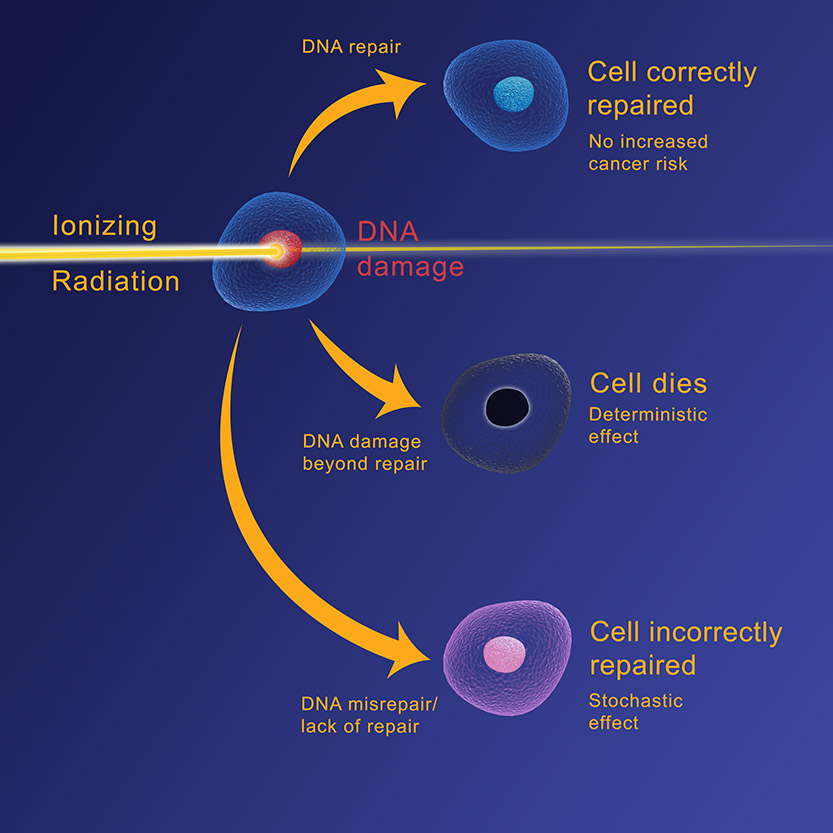
3) The cell incorrectly repairs itself, but it continues to live (stochastic effects)
In some cases, the DNA of the cell may be damaged by radiation, but the damage does not kill the cell. The cell may continue to live and even reproduce itself, but the cell and its descendents may no longer function properly and may disrupt the function of other cells. The probability of this type of detrimental effect is proportionate to the dose and it is called a stochastic effect – when there is a statistical probability that the effects of exposure will occur. In such cases, the likelihood of the effects increases as the dose increases. However, the timing of the effects or their severity does not depend on the dose.
This process happens all the time in everyone. In fact, people are exposed to about 15,000 such events every second of every day. Sometimes, the cell structure changes because it repairs itself improperly. This alteration could have no further effect, or the effect could show up later in life. Cancer and hereditary effects may or may not take place.
Epidemiological evidence
Studies on survivors of the atomic bombings of the cities of Hiroshima and Nagasaki in 1945 indicate that the principal long-term effects of radiation exposure have been an increase in the frequency of cancer and leukemia.
Similar results have been found in:
- people who have been medically treated or diagnosed with radiation;
- early uranium mine workers;
- workers who manufactured atomic weapons;
- people exposed to radiation as a result of the Chernobyl nuclear accident; and,
- people exposed to radon gas in their homes.
Studies have shown that radiation will increase the frequency of some cancers that already occur naturally and that this increase is proportionate to the radiation dose – i.e., the greater the dose, the greater the risk of cancer. However, studies to date have not been able to show any excess cancers or other diseases in people chronically exposed to radiation at doses lower than about 100 mSv. (The lowest dose for excess cancers in atomic bomb survivors was about 80 mSv).
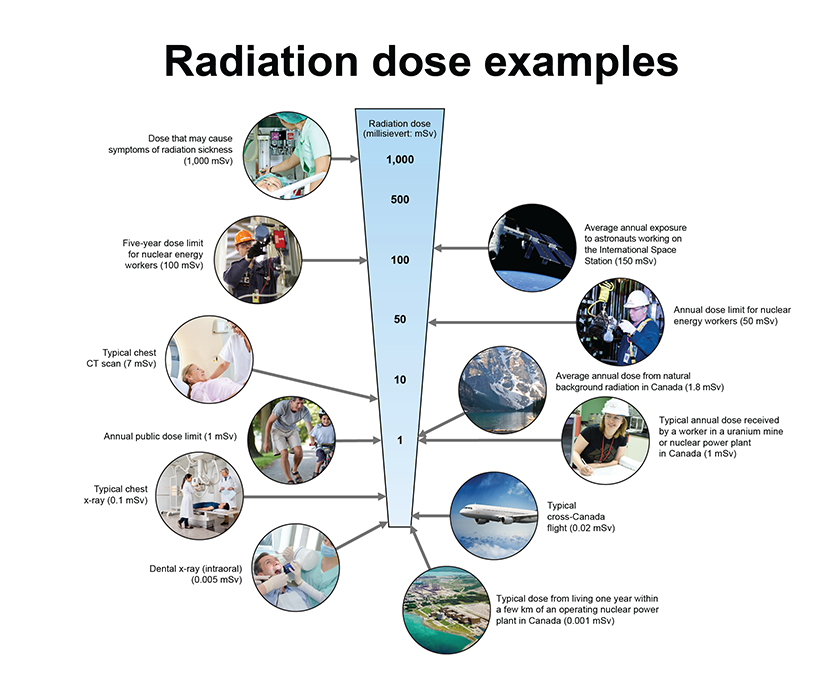
Text version
Radiation dose examples. Figure showing examples of typical doses received and dose limits for workers and the public ranging from 0.001 mSv to 1,000 mSv. The typical annual dose from living within a few kilometers of a nuclear power plant is 0.001 mSv. An intraoral dental x-ray is 0.005 mSv. A typical cross-Canada flight is 0.02 mSv. A typical chest x-ray is 0.1 mSv. The annual public dose limit is 1 mSv. A typical annual dose received by a worker in a uranium mine or nuclear power plant in Canada is around 1 mSv. The average annual dose from natural background radiation in Canada is 1.8 mSv. A typical chest CT scan is 7 mSv. The annual dose limit for nuclear energy workers is 50 mSv. The five-year dose limit for nuclear energy workers is 100 mSv. The average annual exposure to astronauts working on the International Space Station is 150 mSv. A dose that may cause symptoms of radiation sickness is around 1000 mSv.
There could be a number of reasons why we cannot see radiation effects below 100 mSv. One reason is that there is a dose threshold below which no cancers are caused. Another reason, supported by the National Academies “Biological Effects of Ionizing Radiation” (BEIR) studies), and the International Commission on Radiological Protection (ICRP), and UNSCEAR is that while cancer can occur as a result of low doses of radiation, the incidence of radiation-induced cancer is so low that it cannot be distinguished from the natural occurrence of the same cancer. Scientists continue to try to detect effects of radiation at low doses to provide proof either way. In the meantime, the CNSC and other regulatory bodies take a cautious approach and assume that all radiation exposure carries some risk.
Most people who showed health effects in studies were exposed to relatively high doses (greater than 100 mSv) delivered in a very short period of time. This is known as “acute” exposure. Normally, workers and members of the public exposed to radiation from the nuclear industry receive much lower doses over a much longer period of time (years as opposed to seconds). This is known as “chronic” exposure. Acute radiation exposures are estimated to be about 1.5 to 2 times more effective in causing health effects.
Radiation-induced hereditary effects
Genetic damage occurs when the DNA of sperm or egg cells are damaged. This causes a harmful characteristic that is passed on from one generation to the next. Animal studies, such as those conducted on fruit flies by Hermann J. Muller in 1926, showed that radiation will cause genetic mutations. However, to date there have been no known genetic effects in humans caused by radiation. This includes studies involving some 30,000 children of survivors of the atomic bombings of the cities of Hiroshima and Nagasaki in Japan in 1945 (BEIR VII) UNSCEAR 2001 Report).
Dose limits
To set dose limits, the CNSC has largely adopted the recommendations of the International Committee on Radiological Protection (ICRP).
ICRP has calculated the probability of a fatal cancer by relying mainly on the assessment of radiation effects by scientific bodies such as the United Nations Scientific Committee on the Effects of Atomic Radiation (UNSCEAR) and BEIR (National Academy of Sciences, Biological Effects of Ionizing Radiation).
The ICRP then determined what they call the overall “detriment” of radiation exposure. This includes:
- the probability of inducing a fatal cancer;
- the chance of a non-fatal cancer occurring;
- the chance of severe hereditary effects; and,
- the length of life loss if the harm occurs (ICRP 103).
Using all these risks, the ICRP has calculated an overall detriment of 0.042 (4.2%) per sievert for adult workers and 0.057 (5.7%) per sievert for the whole population (ICRP 103).
Dose limits are set at a detriment level above which the consequences would be widely regarded as unacceptable (S24, ICRP 103). However, as a prudent measure, it is assumed that every exposure to radiation carries some risk, even if under the dose limit, so there is a regulatory requirement to reduce all doses as low as reasonably achievable, social and economic factors being taken into account. This is commonly referred to as the ALARA principle.
The Linear No-Threshold model (LNT) is a risk model used internationally by most health agencies and nuclear regulators to set dose limits for workers and members of the public. This model plays a key role in how the CNSC approaches radiation protection. The LNT conservatively assumes there is a direct relationship between radiation exposure and cancer rates.
As a result of these regulations, nuclear workers rarely, if ever, approach the dose limit. Doses to which the public are exposed are generally well below one-tenth of the dose limit, or about 0.1 millisieverts, even those living near nuclear facilities.
Page details
- Date modified: